A study to use activated sludge anaerobic combining aerobic for treatment of high salt seafood processing wastewater
Seafood processing operations generate a high strength wastewater, which contain organic
pollutants in soluble, colloidal, particulate form and salt content, up to 30g NaCl/L. This
research aimed to study the effect of salt (NaCl) concentration on the treatment efficiency of
seafood processing wastewater by the use of a laboratory-scale bioreactor, which is operated
in anaerobic combining aerobic system with concentration salt different from 0- 5%. The
results showed that the wastewater from seafood processing with the chemical input
parameters of pH = 7 - 8.5, COD = 2000 mg / L, total nitrate nitrogen = 150 mg / L, NH4+ =
90 mg / L, total phosphorus = 50 mg / L, salt content 3% was treated with anaerobic activated
sludge content of 8000mg/l, 16HRT and combining an aerobic activated sludge content of
6000mg/l, 6HRT, DO=2-4mgO2/l with the acclimatization of 7% bacteria Bacillus velezensis
at high salinity The parameters output wastewater was treated according to standards QCVN
11-MT:2015/BTNMT (column B).
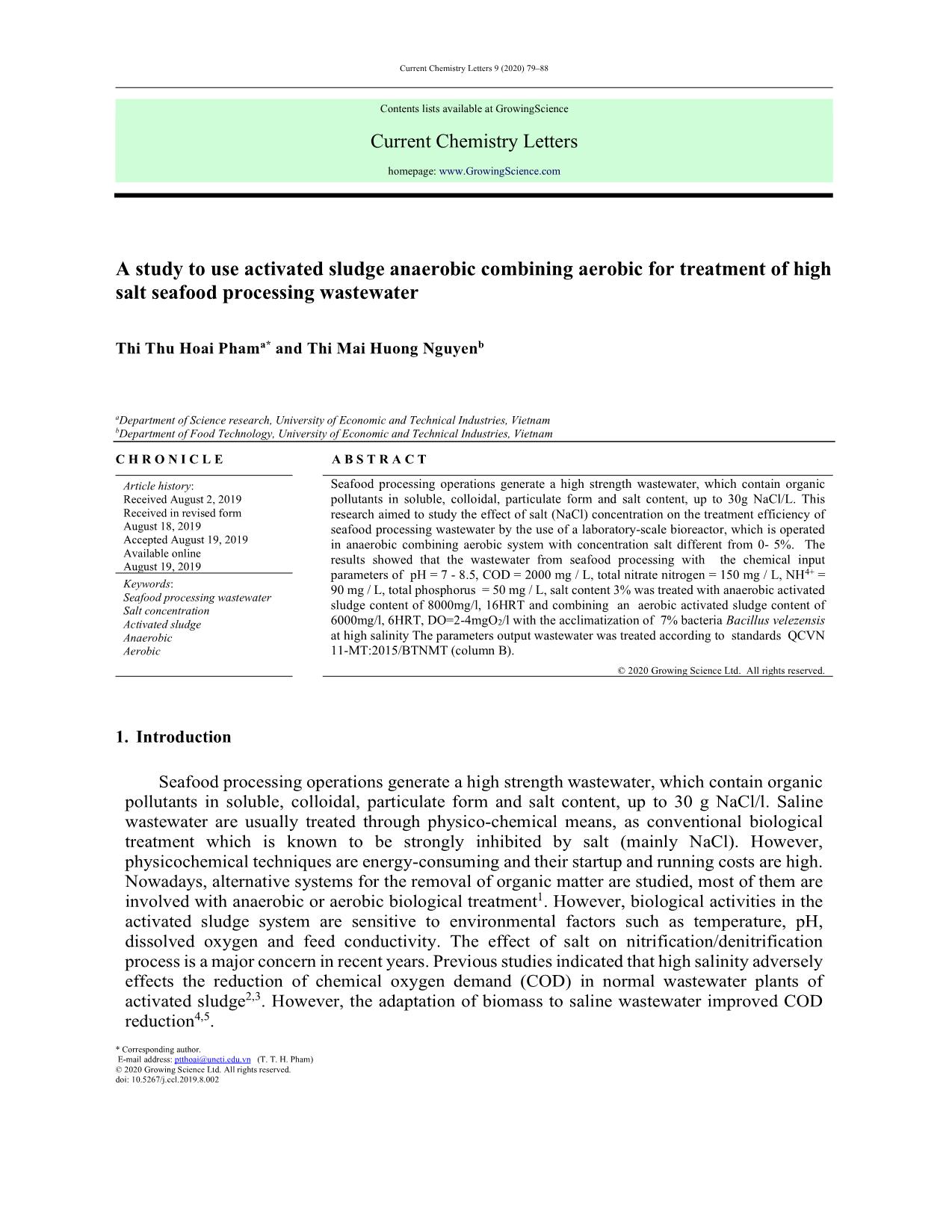
Trang 1
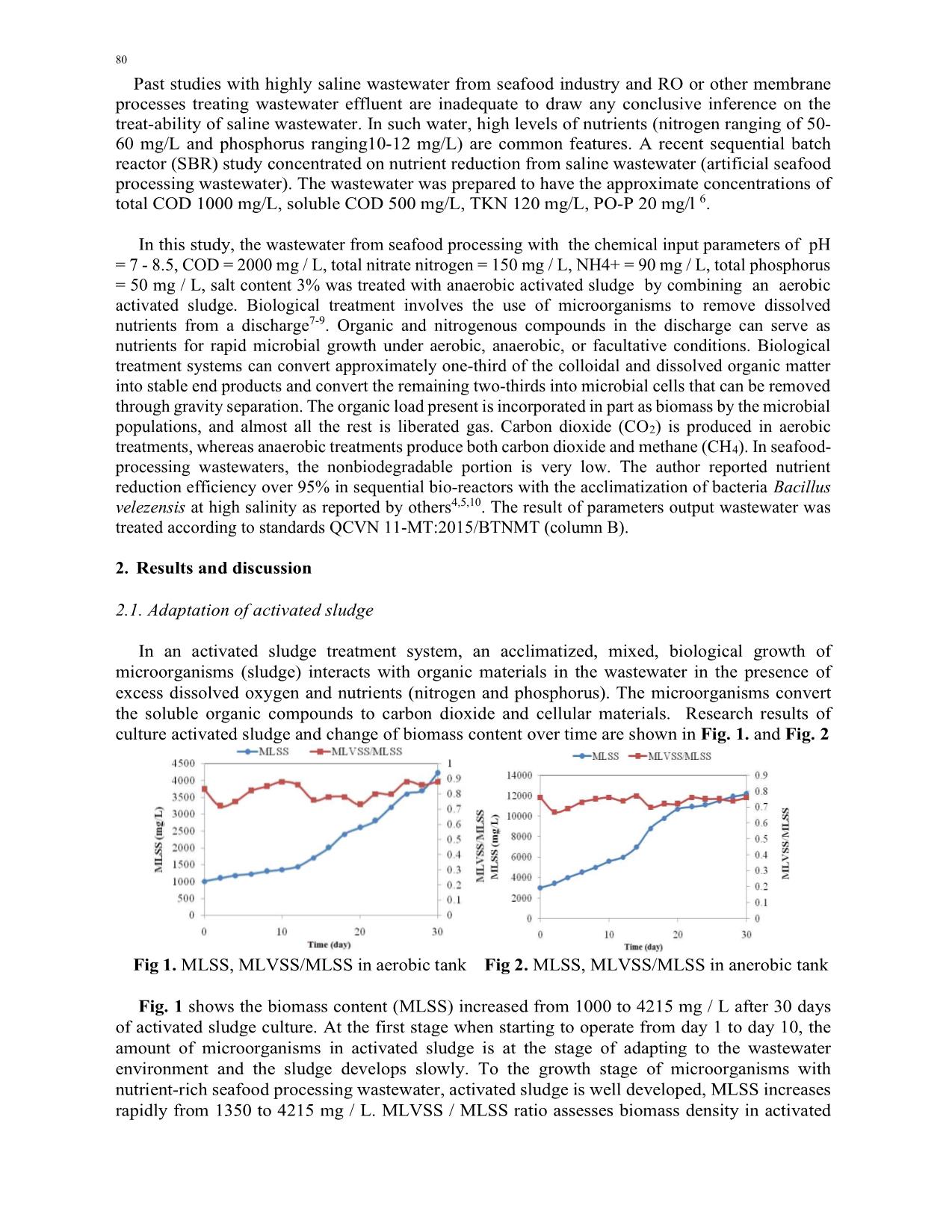
Trang 2
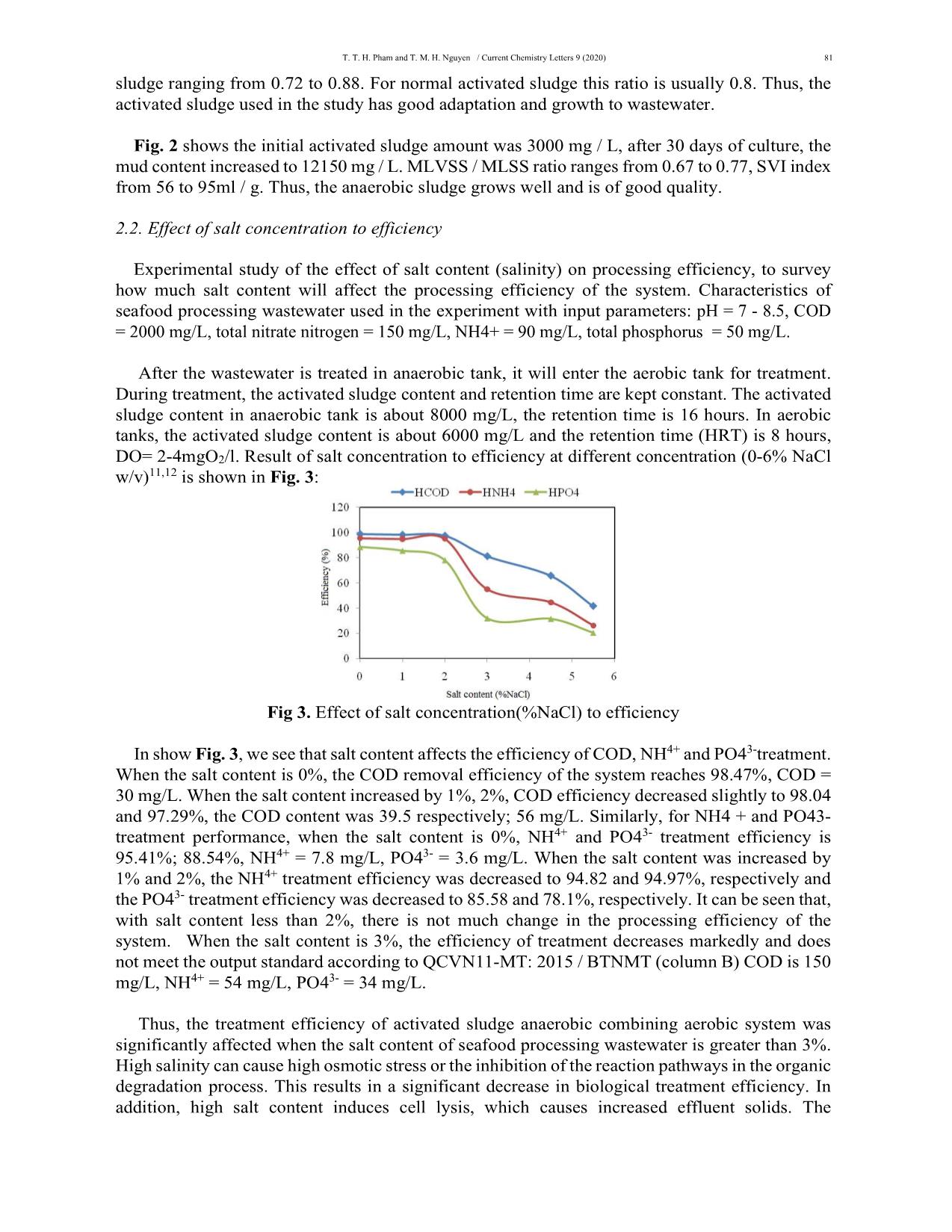
Trang 3
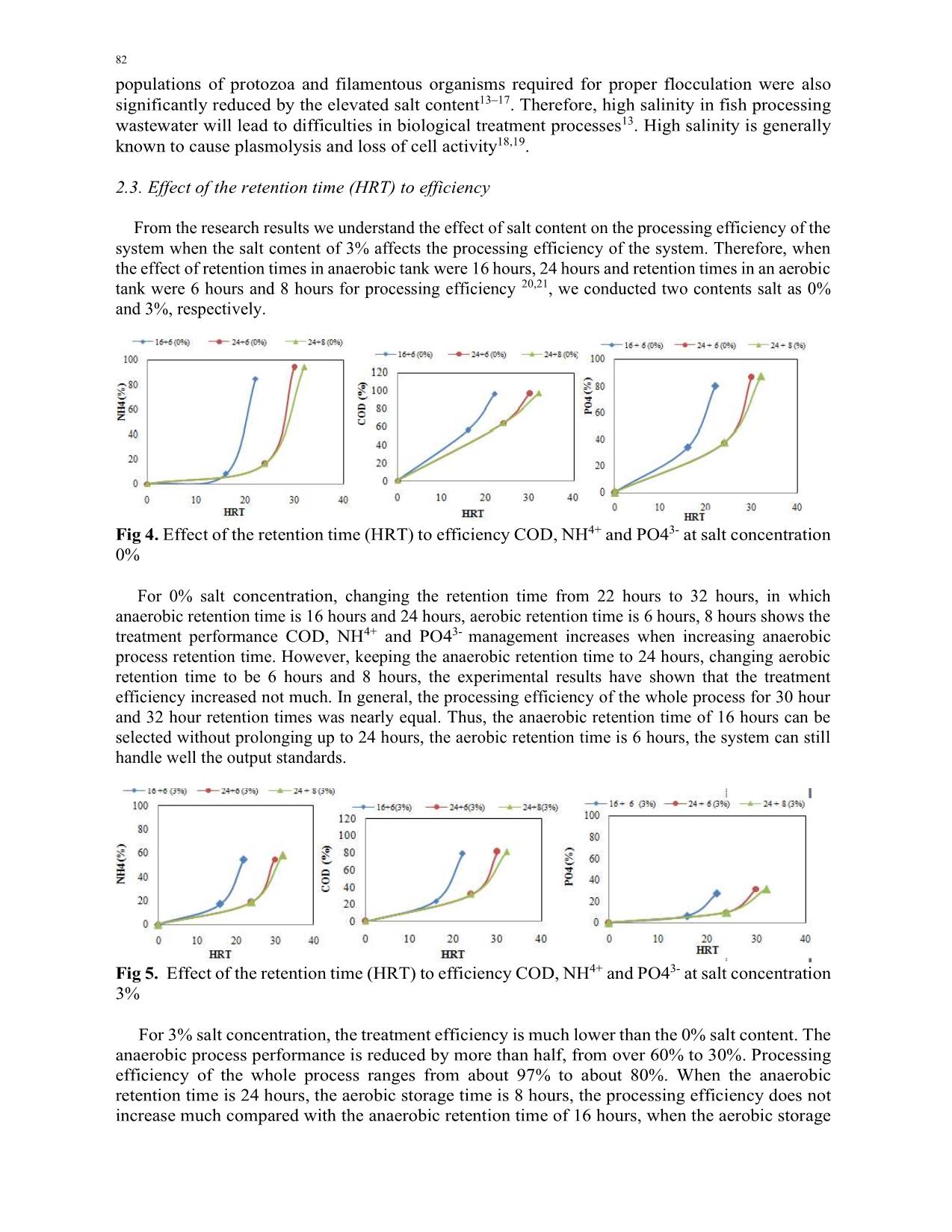
Trang 4
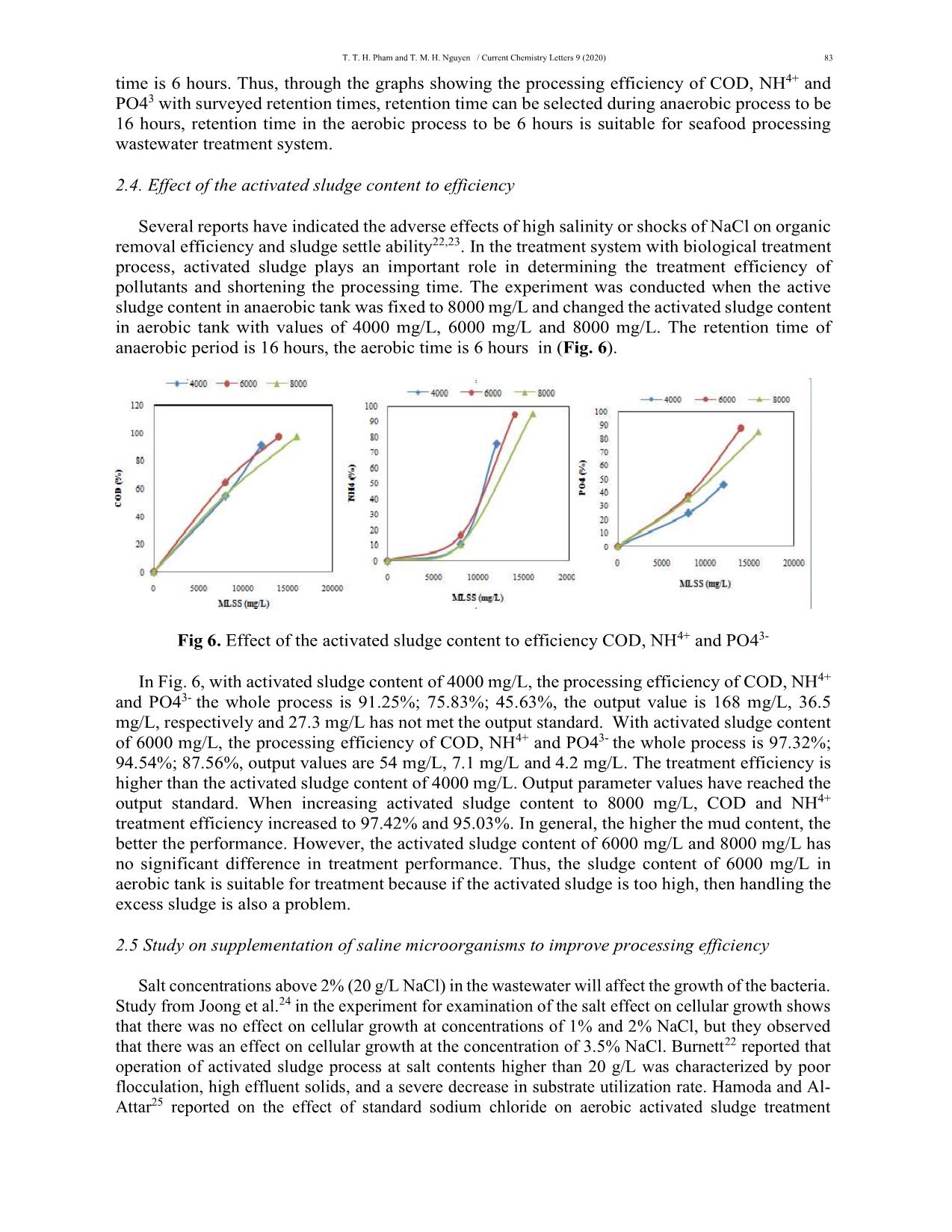
Trang 5
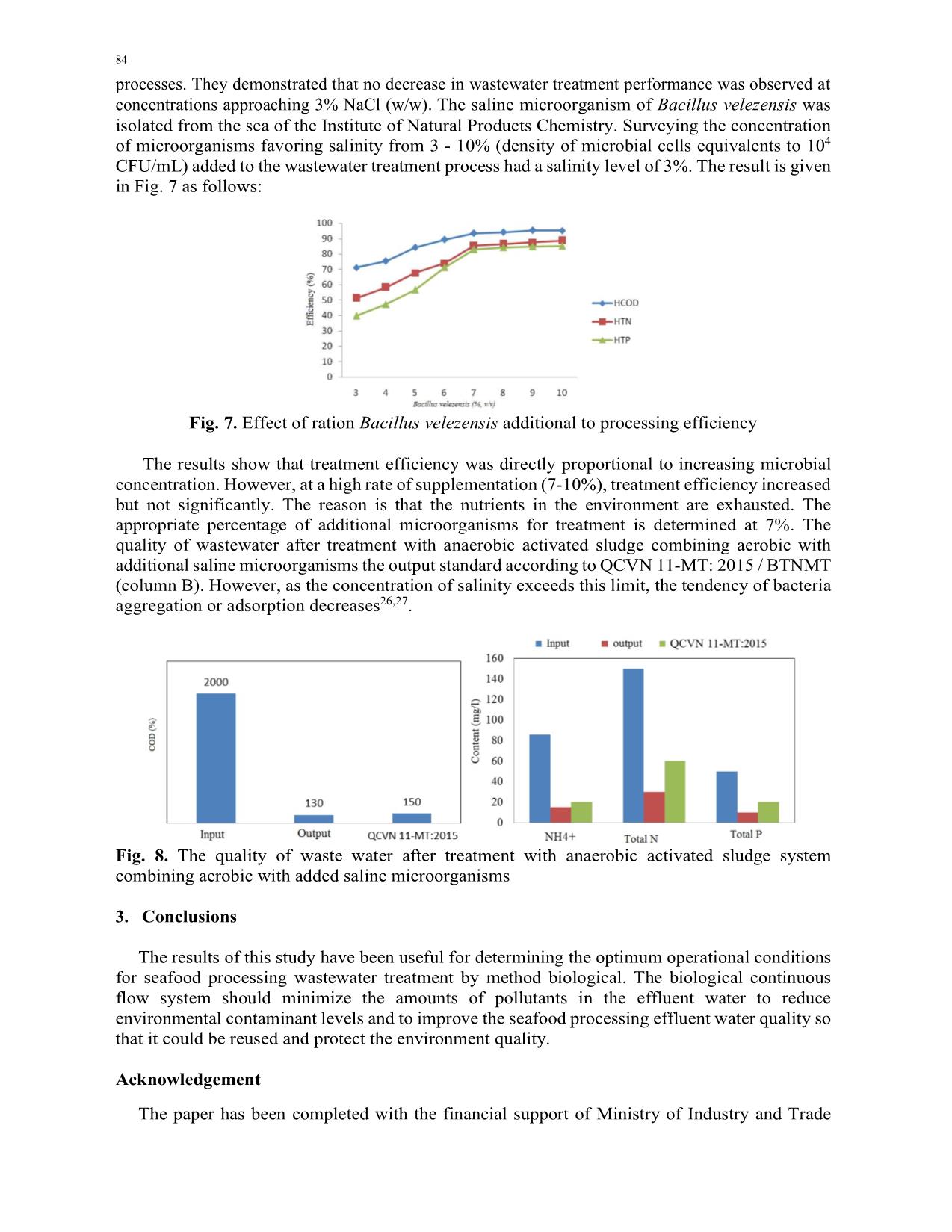
Trang 6
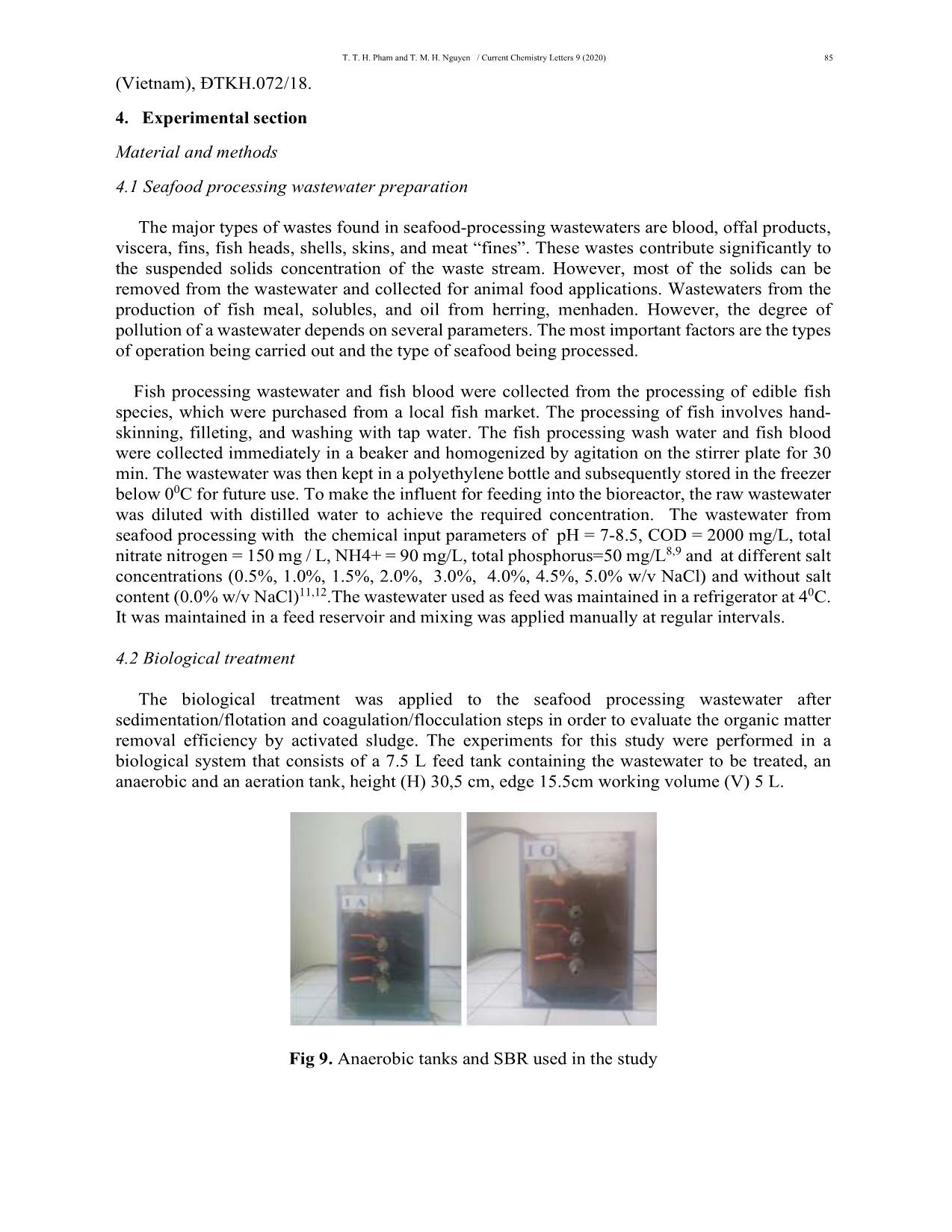
Trang 7
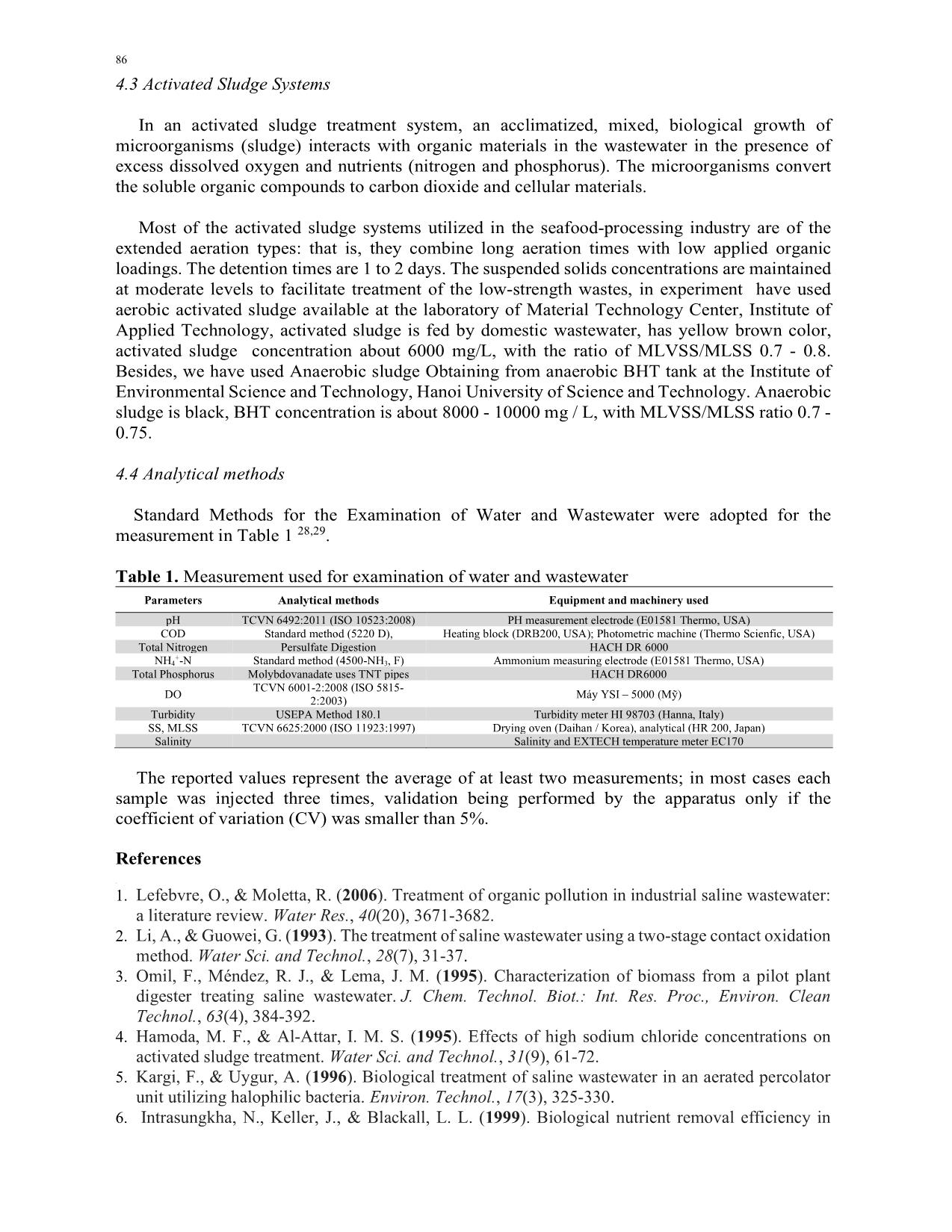
Trang 8
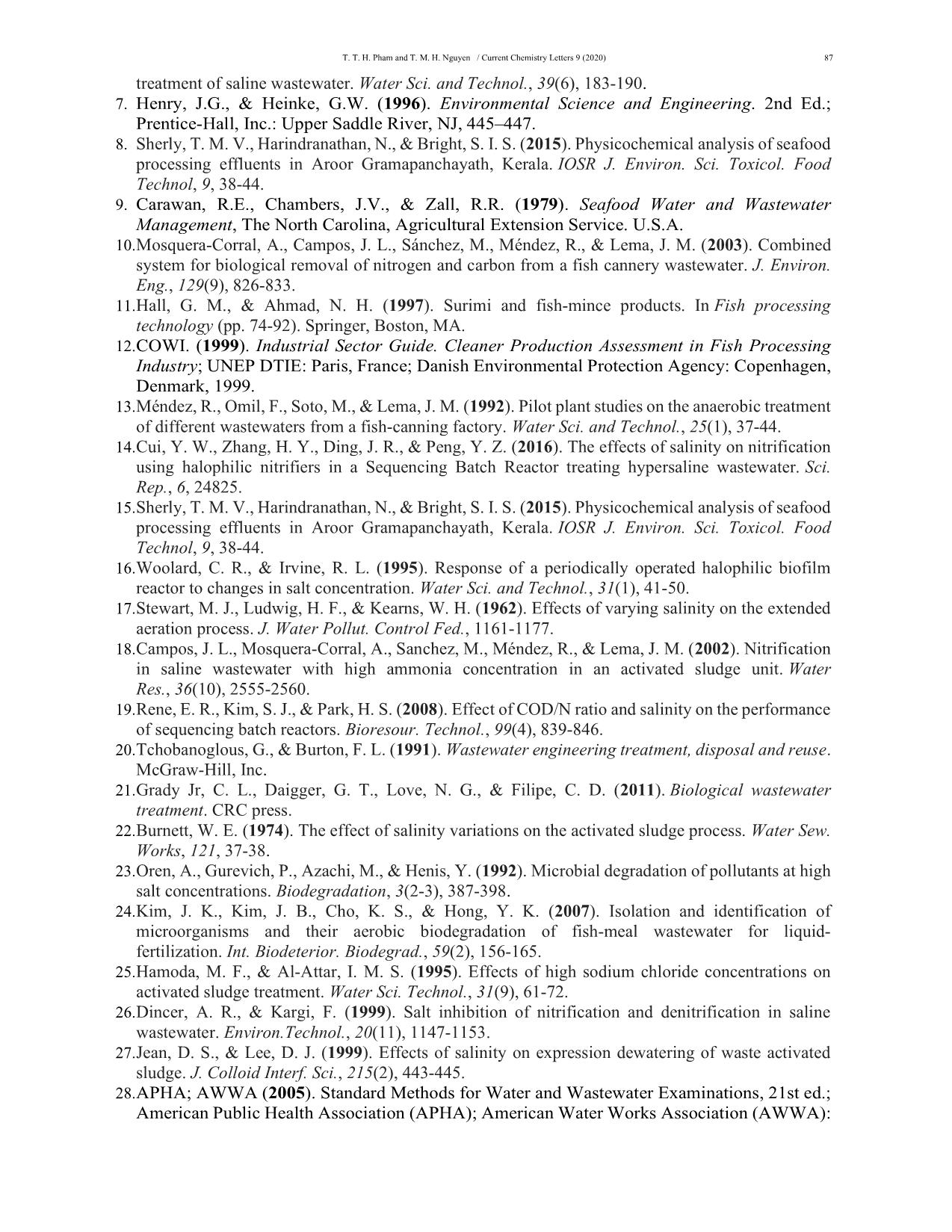
Trang 9
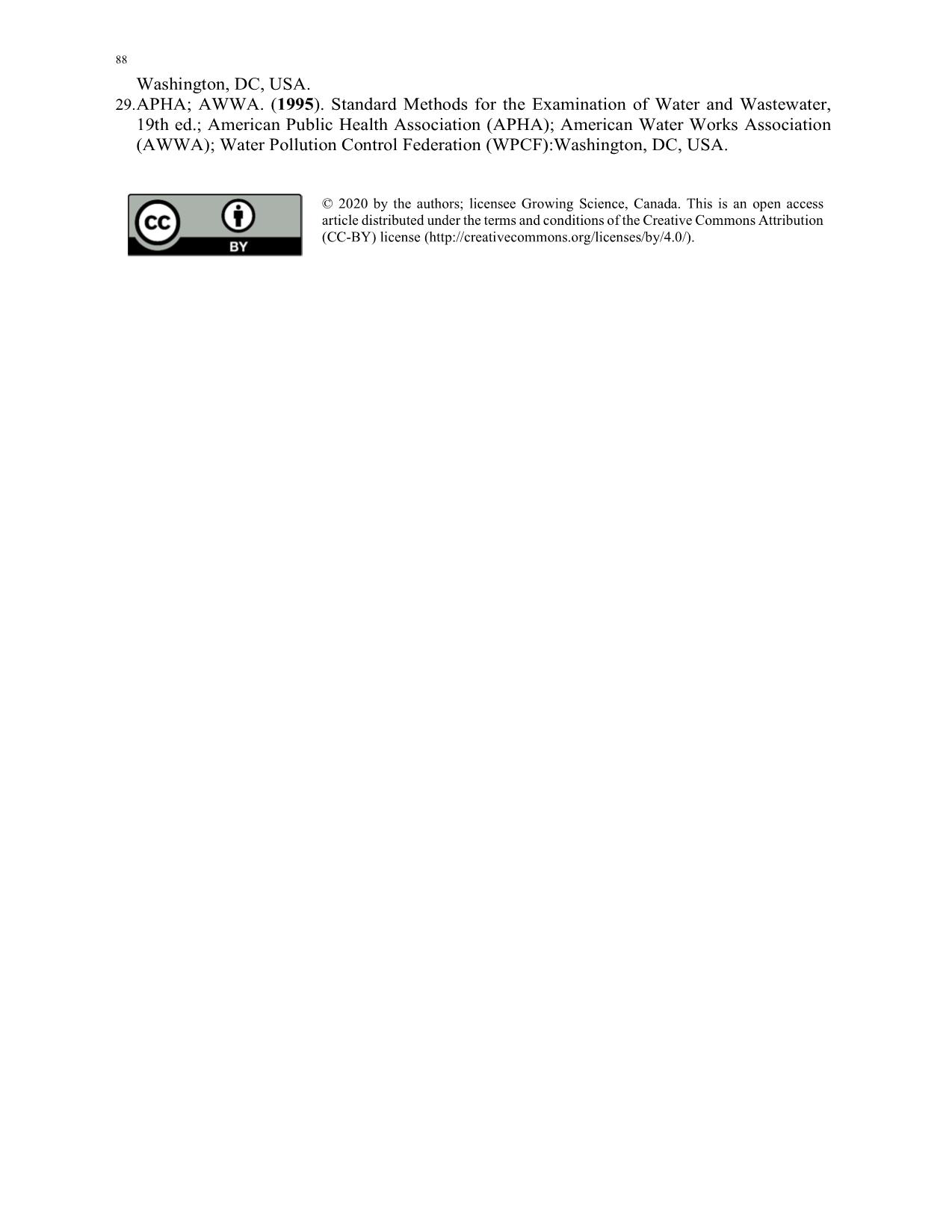
Trang 10
Tóm tắt nội dung tài liệu: A study to use activated sludge anaerobic combining aerobic for treatment of high salt seafood processing wastewater
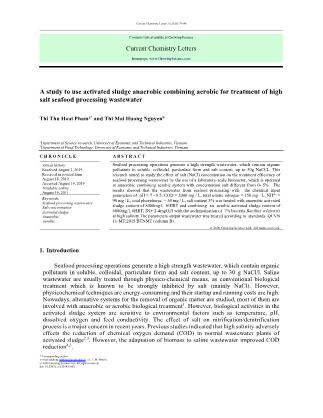
llutants and shortening the processing time. The experiment was conducted when the active sludge content in anaerobic tank was fixed to 8000 mg/L and changed the activated sludge content in aerobic tank with values of 4000 mg/L, 6000 mg/L and 8000 mg/L. The retention time of anaerobic period is 16 hours, the aerobic time is 6 hours in (Fig. 6). Fig 6. Effect of the activated sludge content to efficiency COD, NH4+ and PO43- In Fig. 6, with activated sludge content of 4000 mg/L, the processing efficiency of COD, NH4+ and PO43- the whole process is 91.25%; 75.83%; 45.63%, the output value is 168 mg/L, 36.5 mg/L, respectively and 27.3 mg/L has not met the output standard. With activated sludge content of 6000 mg/L, the processing efficiency of COD, NH4+ and PO43- the whole process is 97.32%; 94.54%; 87.56%, output values are 54 mg/L, 7.1 mg/L and 4.2 mg/L. The treatment efficiency is higher than the activated sludge content of 4000 mg/L. Output parameter values have reached the output standard. When increasing activated sludge content to 8000 mg/L, COD and NH4+ treatment efficiency increased to 97.42% and 95.03%. In general, the higher the mud content, the better the performance. However, the activated sludge content of 6000 mg/L and 8000 mg/L has no significant difference in treatment performance. Thus, the sludge content of 6000 mg/L in aerobic tank is suitable for treatment because if the activated sludge is too high, then handling the excess sludge is also a problem. 2.5 Study on supplementation of saline microorganisms to improve processing efficiency Salt concentrations above 2% (20 g/L NaCl) in the wastewater will affect the growth of the bacteria. Study from Joong et al.24 in the experiment for examination of the salt effect on cellular growth shows that there was no effect on cellular growth at concentrations of 1% and 2% NaCl, but they observed that there was an effect on cellular growth at the concentration of 3.5% NaCl. Burnett22 reported that operation of activated sludge process at salt contents higher than 20 g/L was characterized by poor flocculation, high effluent solids, and a severe decrease in substrate utilization rate. Hamoda and Al- Attar25 reported on the effect of standard sodium chloride on aerobic activated sludge treatment 84 processes. They demonstrated that no decrease in wastewater treatment performance was observed at concentrations approaching 3% NaCl (w/w). The saline microorganism of Bacillus velezensis was isolated from the sea of the Institute of Natural Products Chemistry. Surveying the concentration of microorganisms favoring salinity from 3 - 10% (density of microbial cells equivalents to 104 CFU/mL) added to the wastewater treatment process had a salinity level of 3%. The result is given in Fig. 7 as follows: Fig. 7. Effect of ration Bacillus velezensis additional to processing efficiency The results show that treatment efficiency was directly proportional to increasing microbial concentration. However, at a high rate of supplementation (7-10%), treatment efficiency increased but not significantly. The reason is that the nutrients in the environment are exhausted. The appropriate percentage of additional microorganisms for treatment is determined at 7%. The quality of wastewater after treatment with anaerobic activated sludge combining aerobic with additional saline microorganisms the output standard according to QCVN 11-MT: 2015 / BTNMT (column B). However, as the concentration of salinity exceeds this limit, the tendency of bacteria aggregation or adsorption decreases26,27. Fig. 8. The quality of waste water after treatment with anaerobic activated sludge system combining aerobic with added saline microorganisms 3. Conclusions The results of this study have been useful for determining the optimum operational conditions for seafood processing wastewater treatment by method biological. The biological continuous flow system should minimize the amounts of pollutants in the effluent water to reduce environmental contaminant levels and to improve the seafood processing effluent water quality so that it could be reused and protect the environment quality. Acknowledgement The paper has been completed with the financial support of Ministry of Industry and Trade T. T. H. Pham and T. M. H. Nguyen / Current Chemistry Letters 9 (2020) 85 (Vietnam), ĐTKH.072/18. 4. Experimental section Material and methods 4.1 Seafood processing wastewater preparation The major types of wastes found in seafood-processing wastewaters are blood, offal products, viscera, fins, fish heads, shells, skins, and meat “fines”. These wastes contribute significantly to the suspended solids concentration of the waste stream. However, most of the solids can be removed from the wastewater and collected for animal food applications. Wastewaters from the production of fish meal, solubles, and oil from herring, menhaden. However, the degree of pollution of a wastewater depends on several parameters. The most important factors are the types of operation being carried out and the type of seafood being processed. Fish processing wastewater and fish blood were collected from the processing of edible fish species, which were purchased from a local fish market. The processing of fish involves hand- skinning, filleting, and washing with tap water. The fish processing wash water and fish blood were collected immediately in a beaker and homogenized by agitation on the stirrer plate for 30 min. The wastewater was then kept in a polyethylene bottle and subsequently stored in the freezer below 00C for future use. To make the influent for feeding into the bioreactor, the raw wastewater was diluted with distilled water to achieve the required concentration. The wastewater from seafood processing with the chemical input parameters of pH = 7-8.5, COD = 2000 mg/L, total nitrate nitrogen = 150 mg / L, NH4+ = 90 mg/L, total phosphorus=50 mg/L8,9 and at different salt concentrations (0.5%, 1.0%, 1.5%, 2.0%, 3.0%, 4.0%, 4.5%, 5.0% w/v NaCl) and without salt content (0.0% w/v NaCl)11,12.The wastewater used as feed was maintained in a refrigerator at 40C. It was maintained in a feed reservoir and mixing was applied manually at regular intervals. 4.2 Biological treatment The biological treatment was applied to the seafood processing wastewater after sedimentation/flotation and coagulation/flocculation steps in order to evaluate the organic matter removal efficiency by activated sludge. The experiments for this study were performed in a biological system that consists of a 7.5 L feed tank containing the wastewater to be treated, an anaerobic and an aeration tank, height (H) 30,5 cm, edge 15.5cm working volume (V) 5 L. Fig 9. Anaerobic tanks and SBR used in the study 86 4.3 Activated Sludge Systems In an activated sludge treatment system, an acclimatized, mixed, biological growth of microorganisms (sludge) interacts with organic materials in the wastewater in the presence of excess dissolved oxygen and nutrients (nitrogen and phosphorus). The microorganisms convert the soluble organic compounds to carbon dioxide and cellular materials. Most of the activated sludge systems utilized in the seafood-processing industry are of the extended aeration types: that is, they combine long aeration times with low applied organic loadings. The detention times are 1 to 2 days. The suspended solids concentrations are maintained at moderate levels to facilitate treatment of the low-strength wastes, in experiment have used aerobic activated sludge available at the laboratory of Material Technology Center, Institute of Applied Technology, activated sludge is fed by domestic wastewater, has yellow brown color, activated sludge concentration about 6000 mg/L, with the ratio of MLVSS/MLSS 0.7 - 0.8. Besides, we have used Anaerobic sludge Obtaining from anaerobic BHT tank at the Institute of Environmental Science and Technology, Hanoi University of Science and Technology. Anaerobic sludge is black, BHT concentration is about 8000 - 10000 mg / L, with MLVSS/MLSS ratio 0.7 - 0.75. 4.4 Analytical methods Standard Methods for the Examination of Water and Wastewater were adopted for the measurement in Table 1 28,29. Table 1. Measurement used for examination of water and wastewater Parameters Analytical methods Equipment and machinery used pH TCVN 6492:2011 (ISO 10523:2008) PH measurement electrode (E01581 Thermo, USA) COD Standard method (5220 D), Heating block (DRB200, USA); Photometric machine (Thermo Scienfic, USA) Total Nitrogen Persulfate Digestion HACH DR 6000 NH4+-N Standard method (4500-NH3, F) Ammonium measuring electrode (E01581 Thermo, USA) Total Phosphorus Molybdovanadate uses TNT pipes HACH DR6000 DO TCVN 6001-2:2008 (ISO 5815-2:2003) Máy YSI – 5000 (Mỹ) Turbidity USEPA Method 180.1 Turbidity meter HI 98703 (Hanna, Italy) SS, MLSS TCVN 6625:2000 (ISO 11923:1997) Drying oven (Daihan / Korea), analytical (HR 200, Japan) Salinity Salinity and EXTECH temperature meter EC170 The reported values represent the average of at least two measurements; in most cases each sample was injected three times, validation being performed by the apparatus only if the coefficient of variation (CV) was smaller than 5%. References 4 1. Lefebvre, O., & Moletta, R. (2006). Treatment of organic pollution in industrial saline wastewater: a literature review. Water Res., 40(20), 3671-3682. 2. Li, A., & Guowei, G. (1993). The treatment of saline wastewater using a two-stage contact oxidation method. Water Sci. and Technol., 28(7), 31-37. 3. Omil, F., Méndez, R. J., & Lema, J. M. (1995). Characterization of biomass from a pilot plant digester treating saline wastewater. J. Chem. Technol. Biot.: Int. Res. Proc., Environ. Clean Technol., 63(4), 384-392. 4. Hamoda, M. F., & Al-Attar, I. M. S. (1995). Effects of high sodium chloride concentrations on activated sludge treatment. Water Sci. and Technol., 31(9), 61-72. 5. Kargi, F., & Uygur, A. (1996). Biological treatment of saline wastewater in an aerated percolator unit utilizing halophilic bacteria. Environ. Technol., 17(3), 325-330. 6. Intrasungkha, N., Keller, J., & Blackall, L. L. (1999). Biological nutrient removal efficiency in T. T. H. Pham and T. M. H. Nguyen / Current Chemistry Letters 9 (2020) 87 treatment of saline wastewater. Water Sci. and Technol., 39(6), 183-190. 7. Henry, J.G., & Heinke, G.W. (1996). Environmental Science and Engineering. 2nd Ed.; Prentice-Hall, Inc.: Upper Saddle River, NJ, 445–447. 8. Sherly, T. M. V., Harindranathan, N., & Bright, S. I. S. (2015). Physicochemical analysis of seafood processing effluents in Aroor Gramapanchayath, Kerala. IOSR J. Environ. Sci. Toxicol. Food Technol, 9, 38-44. 9. Carawan, R.E., Chambers, J.V., & Zall, R.R. (1979). Seafood Water and Wastewater Management, The North Carolina, Agricultural Extension Service. U.S.A. 10. Mosquera-Corral, A., Campos, J. L., Sánchez, M., Méndez, R., & Lema, J. M. (2003). Combined system for biological removal of nitrogen and carbon from a fish cannery wastewater. J. Environ. Eng., 129(9), 826-833. 11. Hall, G. M., & Ahmad, N. H. (1997). Surimi and fish-mince products. In Fish processing technology (pp. 74-92). Springer, Boston, MA. 12. COWI. (1999). Industrial Sector Guide. Cleaner Production Assessment in Fish Processing Industry; UNEP DTIE: Paris, France; Danish Environmental Protection Agency: Copenhagen, Denmark, 1999. 13. Méndez, R., Omil, F., Soto, M., & Lema, J. M. (1992). Pilot plant studies on the anaerobic treatment of different wastewaters from a fish-canning factory. Water Sci. and Technol., 25(1), 37-44. 14. Cui, Y. W., Zhang, H. Y., Ding, J. R., & Peng, Y. Z. (2016). The effects of salinity on nitrification using halophilic nitrifiers in a Sequencing Batch Reactor treating hypersaline wastewater. Sci. Rep., 6, 24825. 15. Sherly, T. M. V., Harindranathan, N., & Bright, S. I. S. (2015). Physicochemical analysis of seafood processing effluents in Aroor Gramapanchayath, Kerala. IOSR J. Environ. Sci. Toxicol. Food Technol, 9, 38-44. 16. Woolard, C. R., & Irvine, R. L. (1995). Response of a periodically operated halophilic biofilm reactor to changes in salt concentration. Water Sci. and Technol., 31(1), 41-50. 17. Stewart, M. J., Ludwig, H. F., & Kearns, W. H. (1962). Effects of varying salinity on the extended aeration process. J. Water Pollut. Control Fed., 1161-1177. 18. Campos, J. L., Mosquera-Corral, A., Sanchez, M., Méndez, R., & Lema, J. M. (2002). Nitrification in saline wastewater with high ammonia concentration in an activated sludge unit. Water Res., 36(10), 2555-2560. 19. Rene, E. R., Kim, S. J., & Park, H. S. (2008). Effect of COD/N ratio and salinity on the performance of sequencing batch reactors. Bioresour. Technol., 99(4), 839-846. 20. Tchobanoglous, G., & Burton, F. L. (1991). Wastewater engineering treatment, disposal and reuse. McGraw-Hill, Inc. 21. Grady Jr, C. L., Daigger, G. T., Love, N. G., & Filipe, C. D. (2011). Biological wastewater treatment. CRC press. 22. Burnett, W. E. (1974). The effect of salinity variations on the activated sludge process. Water Sew. Works, 121, 37-38. 23. Oren, A., Gurevich, P., Azachi, M., & Henis, Y. (1992). Microbial degradation of pollutants at high salt concentrations. Biodegradation, 3(2-3), 387-398. 24. Kim, J. K., Kim, J. B., Cho, K. S., & Hong, Y. K. (2007). Isolation and identification of microorganisms and their aerobic biodegradation of fish-meal wastewater for liquid- fertilization. Int. Biodeterior. Biodegrad., 59(2), 156-165. 25. Hamoda, M. F., & Al-Attar, I. M. S. (1995). Effects of high sodium chloride concentrations on activated sludge treatment. Water Sci. Technol., 31(9), 61-72. 26. Dincer, A. R., & Kargi, F. (1999). Salt inhibition of nitrification and denitrification in saline wastewater. Environ.Technol., 20(11), 1147-1153. 27. Jean, D. S., & Lee, D. J. (1999). Effects of salinity on expression dewatering of waste activated sludge. J. Colloid Interf. Sci., 215(2), 443-445. 28. APHA; AWWA (2005). Standard Methods for Water and Wastewater Examinations, 21st ed.; American Public Health Association (APHA); American Water Works Association (AWWA): 88 Washington, DC, USA. 29. APHA; AWWA. (1995). Standard Methods for the Examination of Water and Wastewater, 19th ed.; American Public Health Association (APHA); American Water Works Association (AWWA); Water Pollution Control Federation (WPCF):Washington, DC, USA. © 2020 by the authors; licensee Growing Science, Canada. This is an open access article distributed under the terms and conditions of the Creative Commons Attribution (CC-BY) license (
File đính kèm:
a_study_to_use_activated_sludge_anaerobic_combining_aerobic.pdf