Study on transmutation efficiency of the VVER-1000 fuel assembly with different minor actinide compositions
The feasibility of transmutation of minor actinides recycled from the spent nuclear fuel in
the VVER-1000 LEU (low enriched uranium) fuel assembly as burnable poison was examined in our
previous study. However, only the minor actinide vector of the VVER-440 spent fuel was considered.
In this paper, various vectors of minor actinides recycled from the spent fuel of VVER-440, PWR-
1000, and VVER-1000 reactors were therefore employed in the analysis in order to investigate the
minor actinide transmutation efficiency of the VVER-1000 fuel assembly with different minor
actinide compositions. The comparative analysis was conducted for the two models of minor actinide
loading in the LEU fuel assembly: homogeneous mixing in the UGD (Uranium-Gadolinium) pins and
coating a thin layer to the UGD pins. The parameters to be analysed and compared include the
reactivity of the LEU fuel assembly versus burnup and the transmutation of minor actinide nuclides
when loading different minor actinide vectors into the LEU fuel assembly.
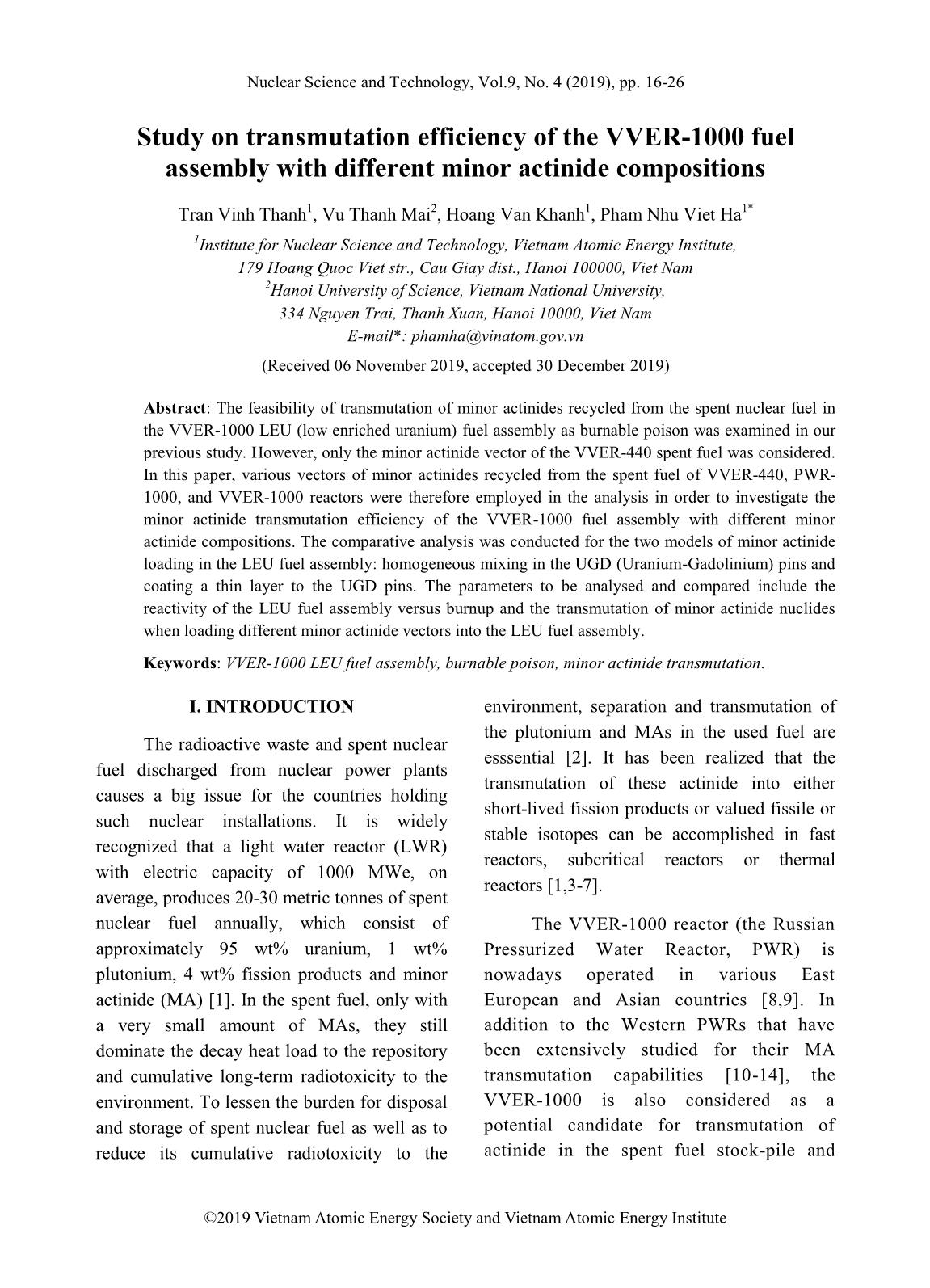
Trang 1
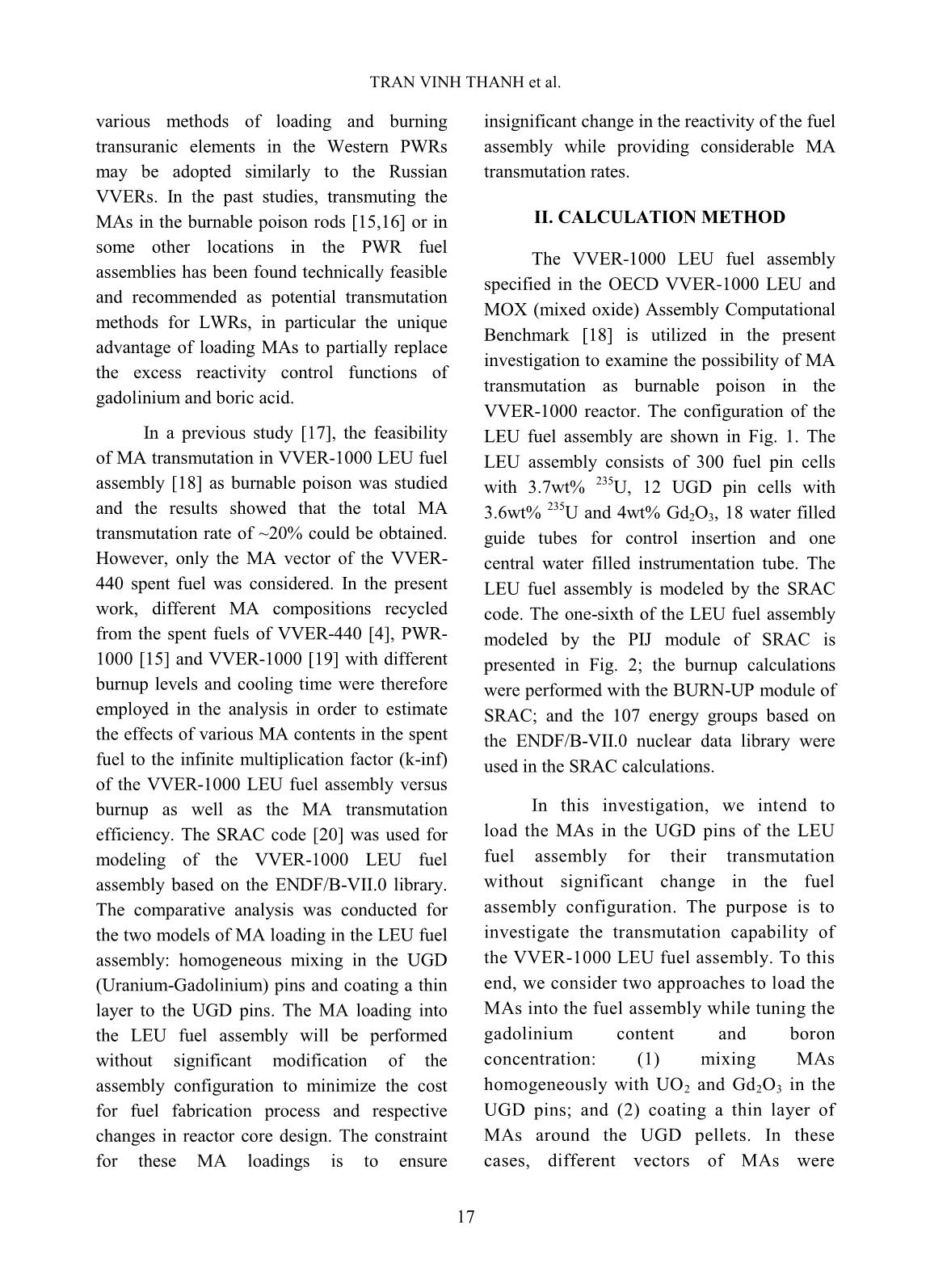
Trang 2
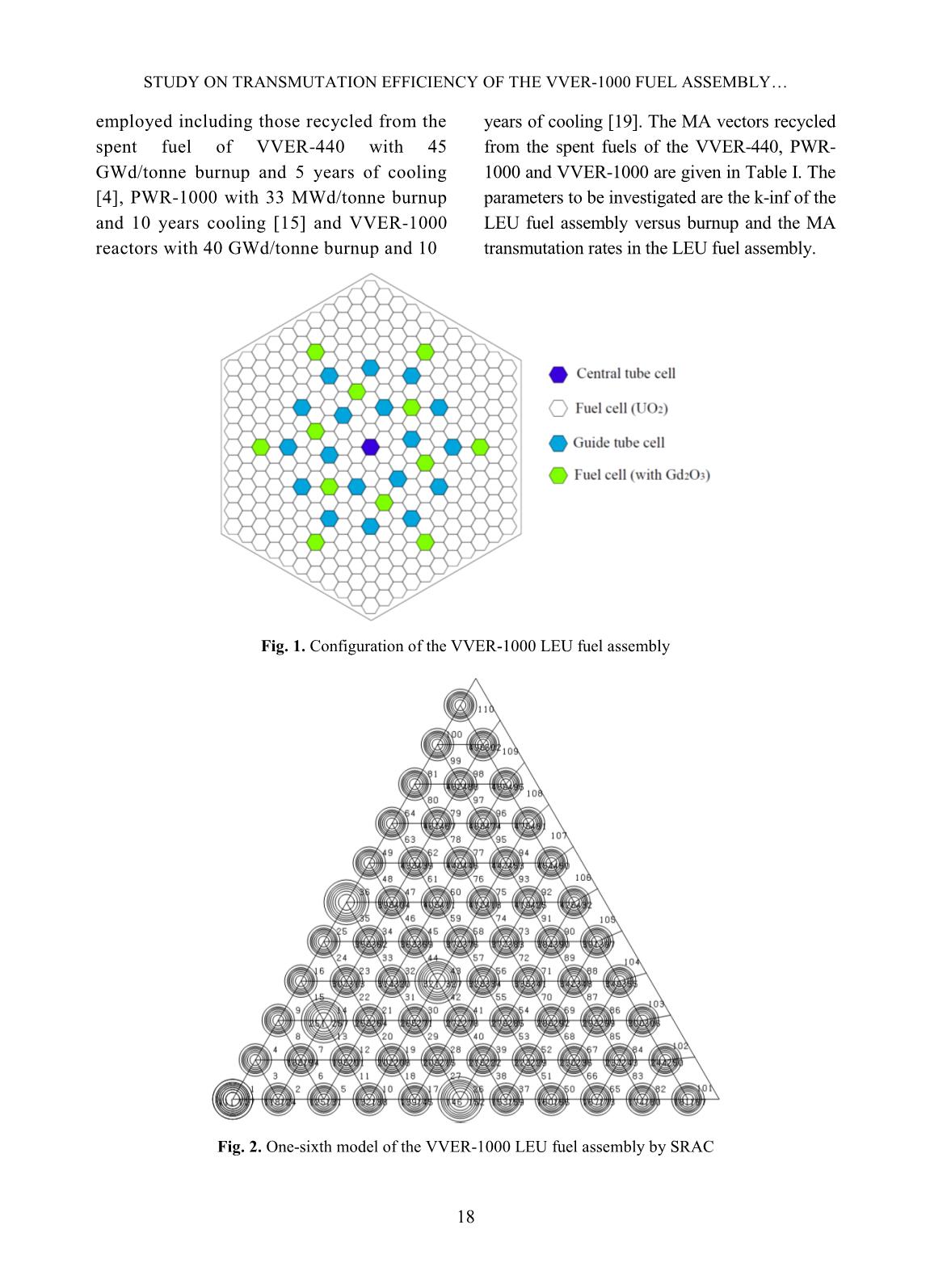
Trang 3
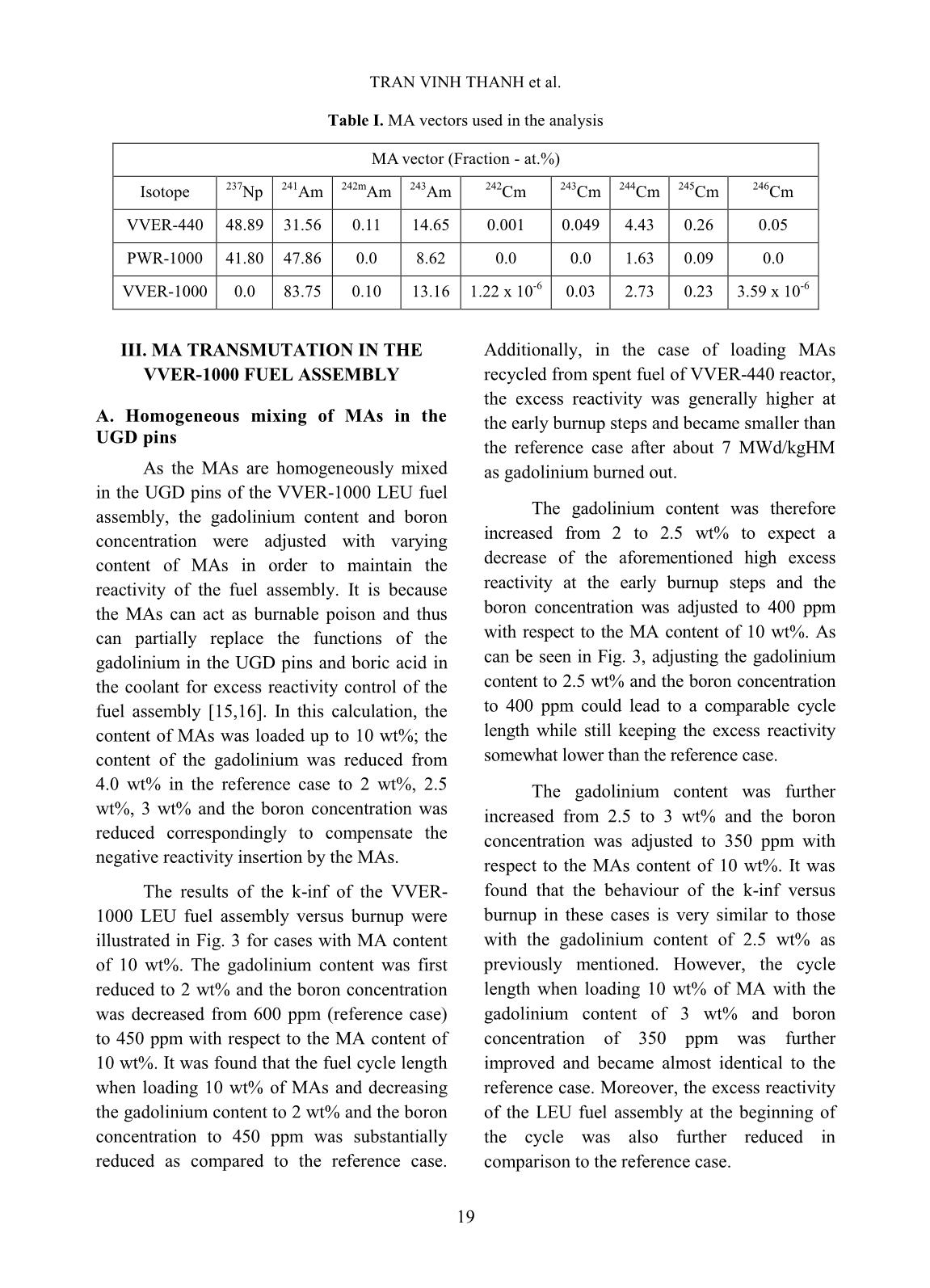
Trang 4
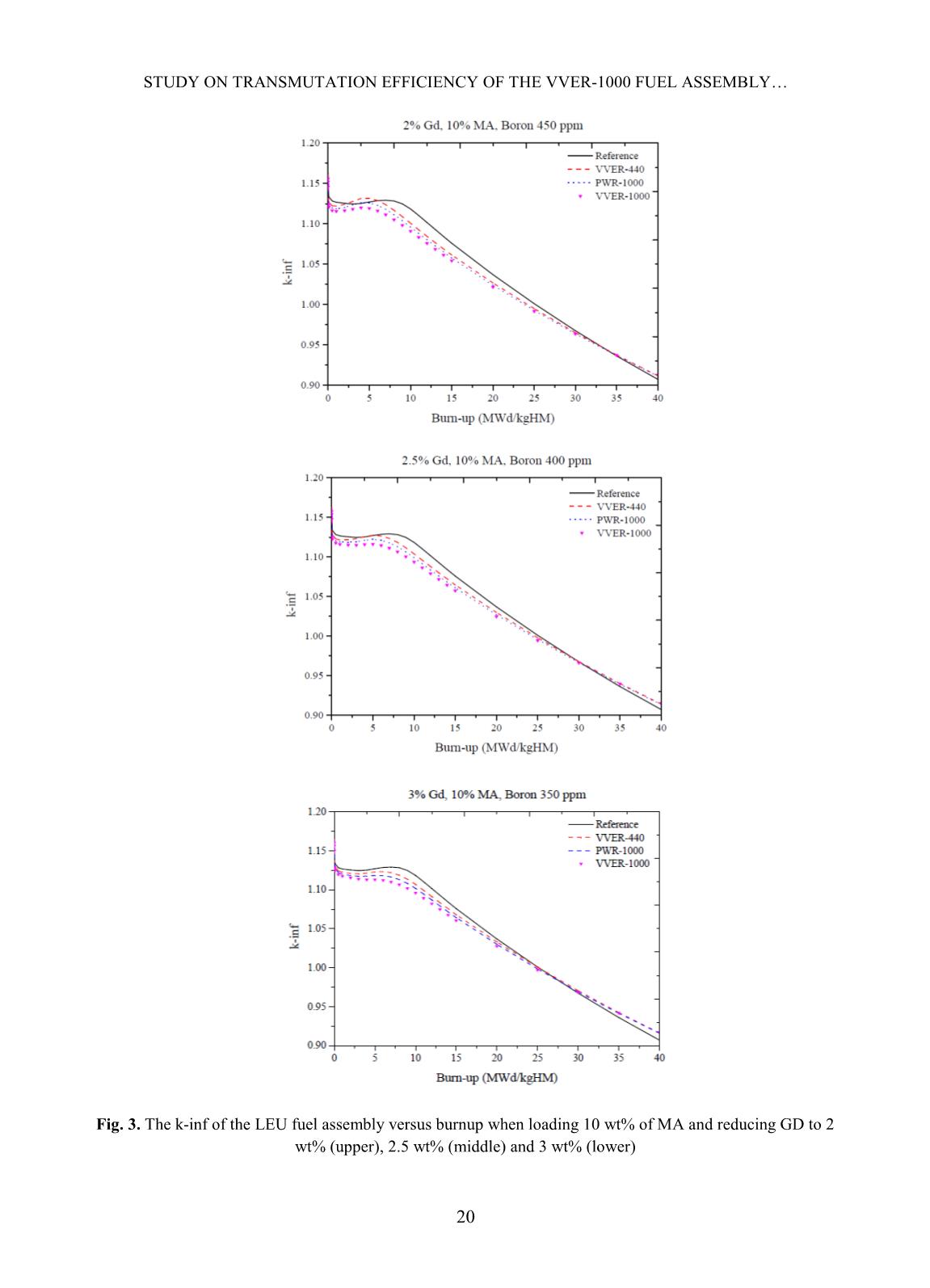
Trang 5
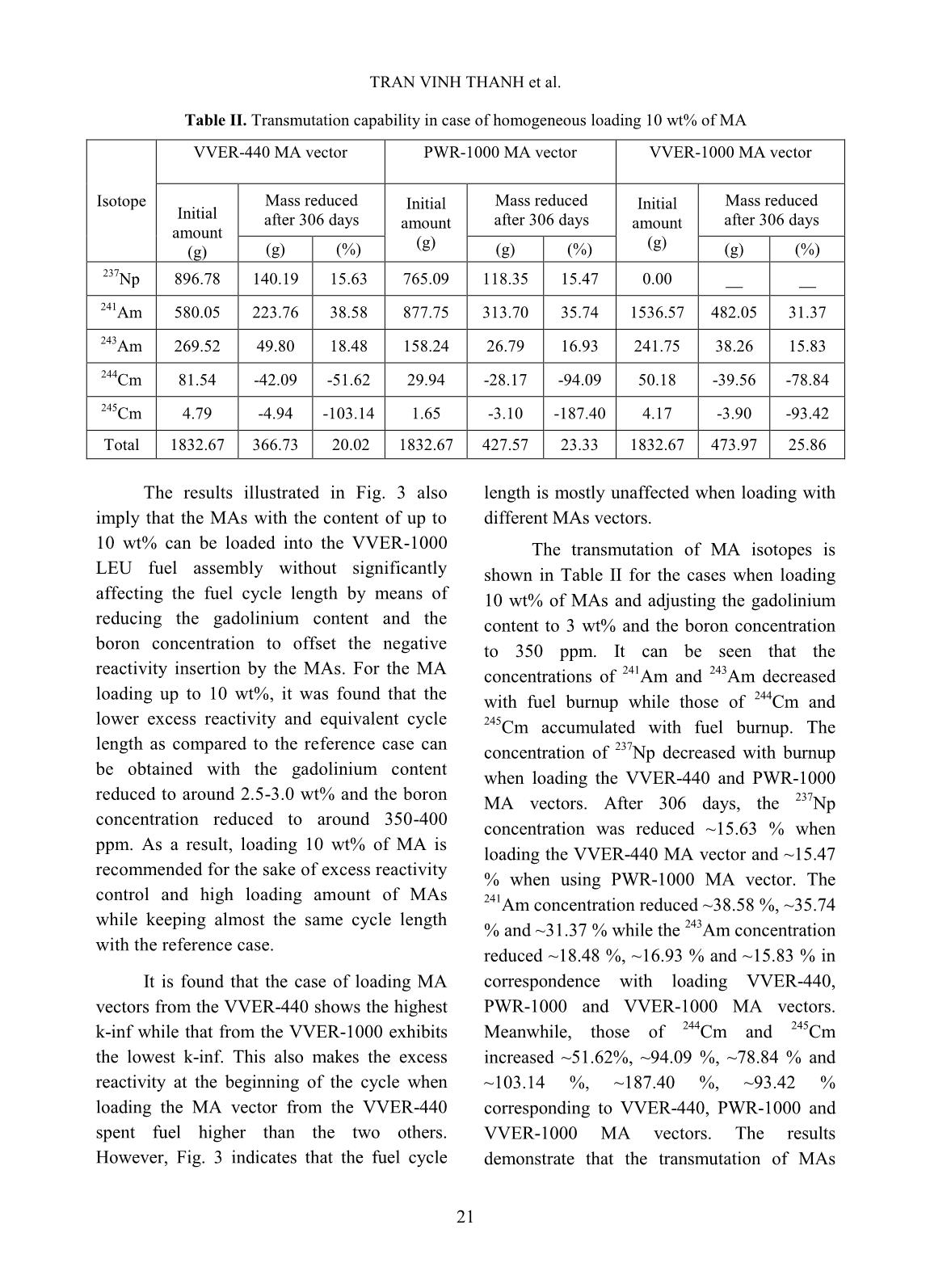
Trang 6
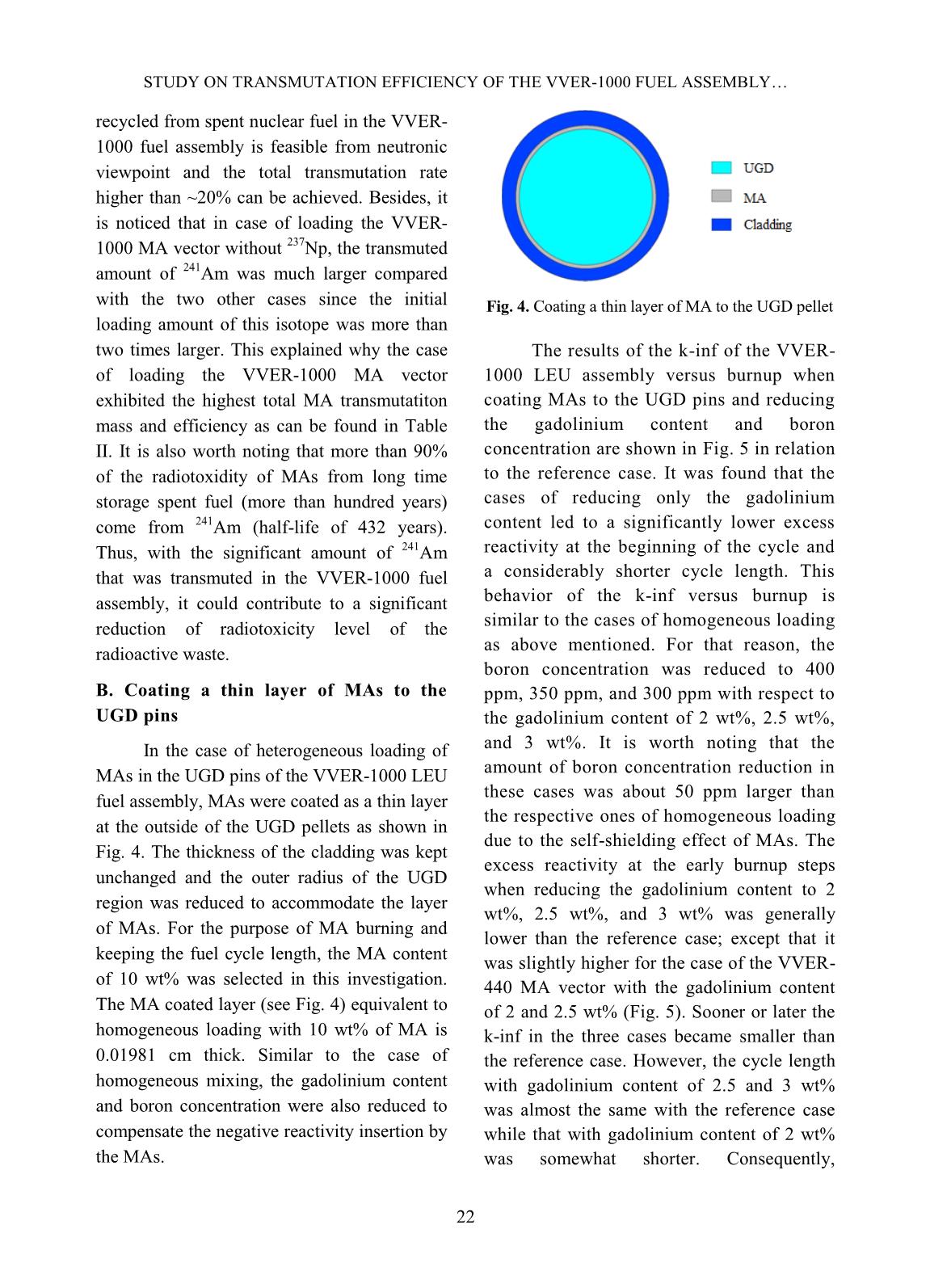
Trang 7
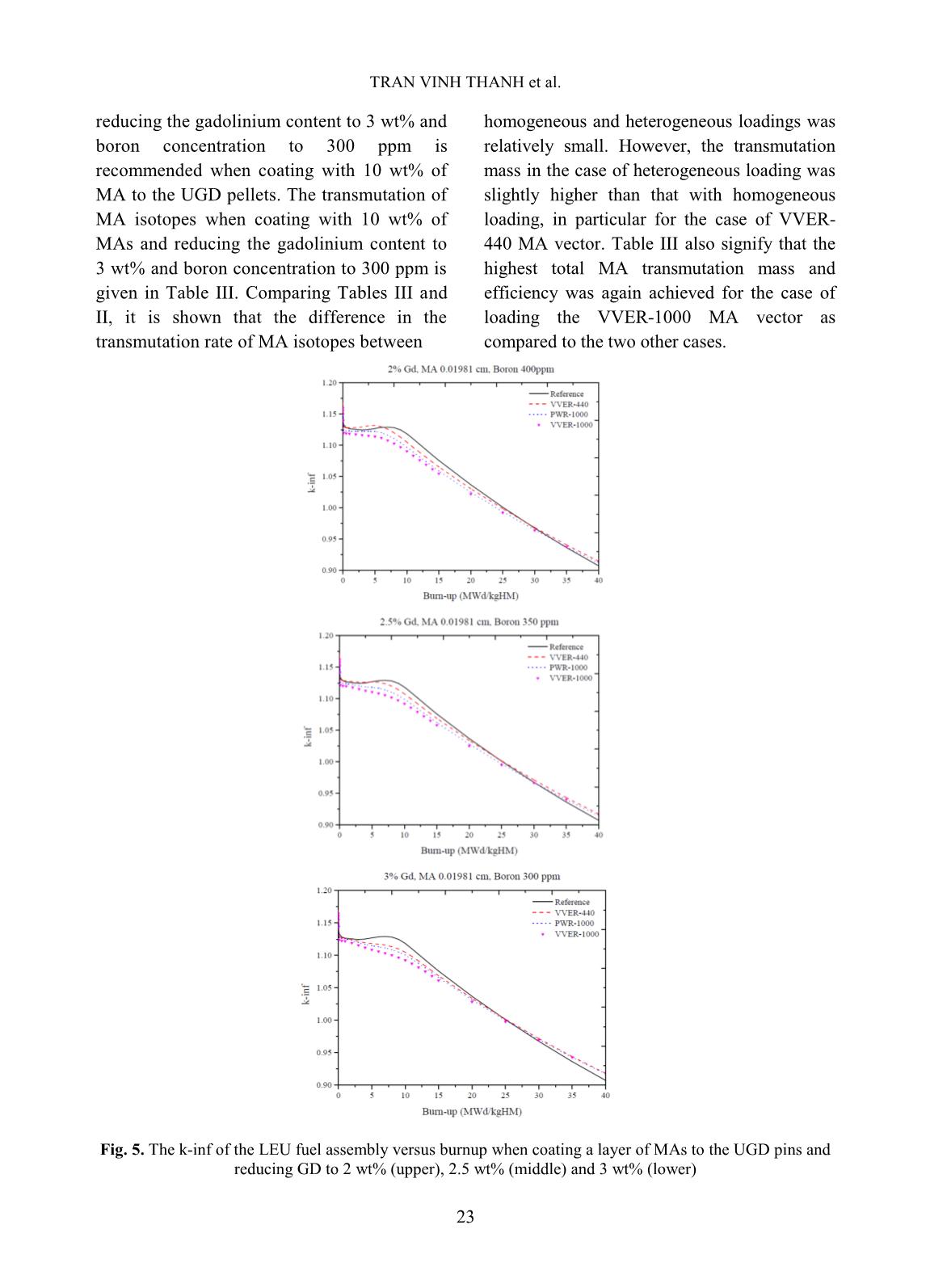
Trang 8
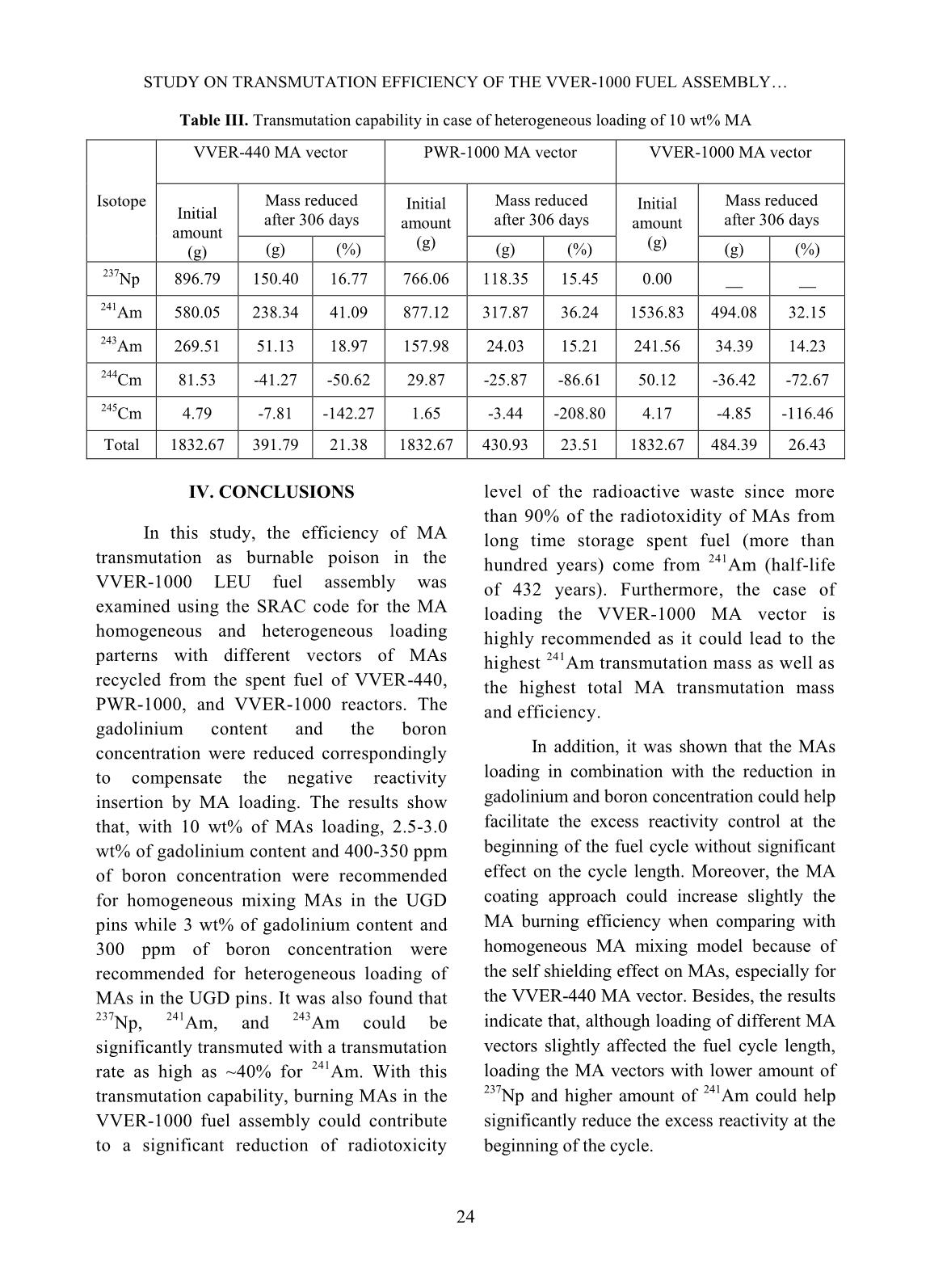
Trang 9
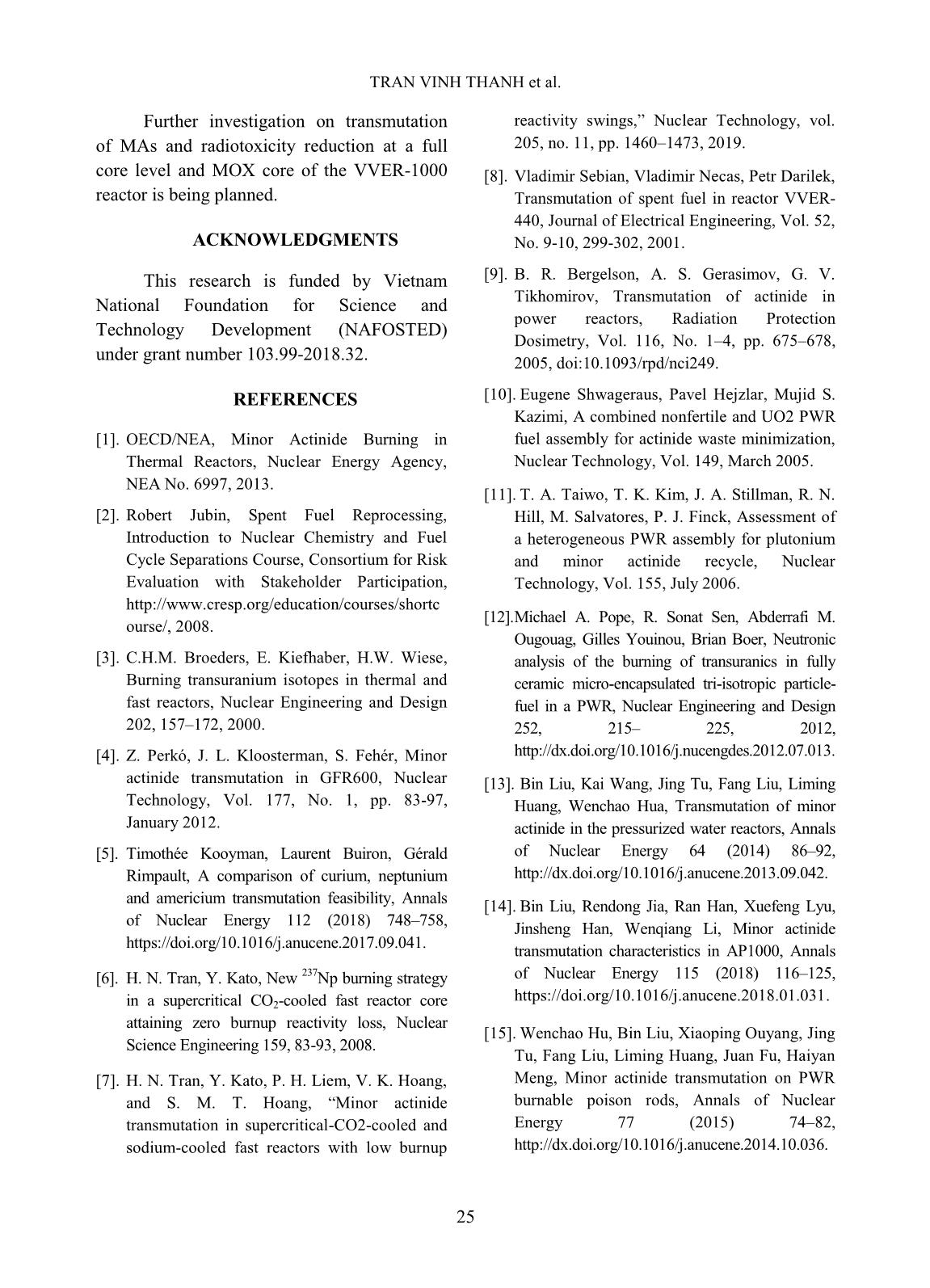
Trang 10
Tải về để xem bản đầy đủ
Tóm tắt nội dung tài liệu: Study on transmutation efficiency of the VVER-1000 fuel assembly with different minor actinide compositions
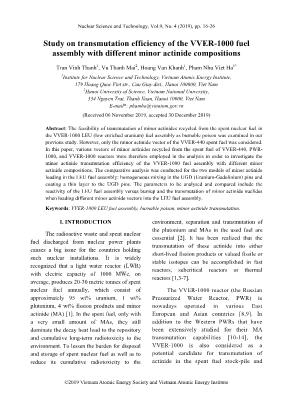
_ 241 Am 580.05 223.76 38.58 877.75 313.70 35.74 1536.57 482.05 31.37 243 Am 269.52 49.80 18.48 158.24 26.79 16.93 241.75 38.26 15.83 244 Cm 81.54 -42.09 -51.62 29.94 -28.17 -94.09 50.18 -39.56 -78.84 245 Cm 4.79 -4.94 -103.14 1.65 -3.10 -187.40 4.17 -3.90 -93.42 Total 1832.67 366.73 20.02 1832.67 427.57 23.33 1832.67 473.97 25.86 The results illustrated in Fig. 3 also imply that the MAs with the content of up to 10 wt% can be loaded into the VVER-1000 LEU fuel assembly without significantly affecting the fuel cycle length by means of reducing the gadolinium content and the boron concentration to offset the negative reactivity insertion by the MAs. For the MA loading up to 10 wt%, it was found that the lower excess reactivity and equivalent cycle length as compared to the reference case can be obtained with the gadolinium content reduced to around 2.5-3.0 wt% and the boron concentration reduced to around 350-400 ppm. As a result, loading 10 wt% of MA is recommended for the sake of excess reactivity control and high loading amount of MAs while keeping almost the same cycle length with the reference case. It is found that the case of loading MA vectors from the VVER-440 shows the highest k-inf while that from the VVER-1000 exhibits the lowest k-inf. This also makes the excess reactivity at the beginning of the cycle when loading the MA vector from the VVER-440 spent fuel higher than the two others. However, Fig. 3 indicates that the fuel cycle length is mostly unaffected when loading with different MAs vectors. The transmutation of MA isotopes is shown in Table II for the cases when loading 10 wt% of MAs and adjusting the gadolinium content to 3 wt% and the boron concentration to 350 ppm. It can be seen that the concentrations of 241 Am and 243 Am decreased with fuel burnup while those of 244 Cm and 245 Cm accumulated with fuel burnup. The concentration of 237 Np decreased with burnup when loading the VVER-440 and PWR-1000 MA vectors. After 306 days, the 237 Np concentration was reduced ~15.63 % when loading the VVER-440 MA vector and ~15.47 % when using PWR-1000 MA vector. The 241 Am concentration reduced ~38.58 %, ~35.74 % and ~31.37 % while the 243 Am concentration reduced ~18.48 %, ~16.93 % and ~15.83 % in correspondence with loading VVER-440, PWR-1000 and VVER-1000 MA vectors. Meanwhile, those of 244 Cm and 245 Cm increased ~51.62%, ~94.09 %, ~78.84 % and ~103.14 %, ~187.40 %, ~93.42 % corresponding to VVER-440, PWR-1000 and VVER-1000 MA vectors. The results demonstrate that the transmutation of MAs STUDY ON TRANSMUTATION EFFICIENCY OF THE VVER-1000 FUEL ASSEMBLY 22 recycled from spent nuclear fuel in the VVER- 1000 fuel assembly is feasible from neutronic viewpoint and the total transmutation rate higher than ~20% can be achieved. Besides, it is noticed that in case of loading the VVER- 1000 MA vector without 237 Np, the transmuted amount of 241 Am was much larger compared with the two other cases since the initial loading amount of this isotope was more than two times larger. This explained why the case of loading the VVER-1000 MA vector exhibited the highest total MA transmutatiton mass and efficiency as can be found in Table II. It is also worth noting that more than 90% of the radiotoxidity of MAs from long time storage spent fuel (more than hundred years) come from 241 Am (half-life of 432 years). Thus, with the significant amount of 241 Am that was transmuted in the VVER-1000 fuel assembly, it could contribute to a significant reduction of radiotoxicity level of the radioactive waste. B. Coating a thin layer of MAs to the UGD pins In the case of heterogeneous loading of MAs in the UGD pins of the VVER-1000 LEU fuel assembly, MAs were coated as a thin layer at the outside of the UGD pellets as shown in Fig. 4. The thickness of the cladding was kept unchanged and the outer radius of the UGD region was reduced to accommodate the layer of MAs. For the purpose of MA burning and keeping the fuel cycle length, the MA content of 10 wt% was selected in this investigation. The MA coated layer (see Fig. 4) equivalent to homogeneous loading with 10 wt% of MA is 0.01981 cm thick. Similar to the case of homogeneous mixing, the gadolinium content and boron concentration were also reduced to compensate the negative reactivity insertion by the MAs. Fig. 4. Coating a thin layer of MA to the UGD pellet The results of the k-inf of the VVER- 1000 LEU assembly versus burnup when coating MAs to the UGD pins and reducing the gadolinium content and boron concentration are shown in Fig. 5 in relation to the reference case. It was found that the cases of reducing only the gadolinium content led to a significantly lower excess reactivity at the beginning of the cycle and a considerably shorter cycle length. This behavior of the k-inf versus burnup is similar to the cases of homogeneous loading as above mentioned. For that reason, the boron concentration was reduced to 400 ppm, 350 ppm, and 300 ppm with respect to the gadolinium content of 2 wt%, 2.5 wt%, and 3 wt%. It is worth noting that the amount of boron concentration reduction in these cases was about 50 ppm larger than the respective ones of homogeneous loading due to the self-shielding effect of MAs. The excess reactivity at the early burnup steps when reducing the gadolinium content to 2 wt%, 2.5 wt%, and 3 wt% was generally lower than the reference case; except that it was slightly higher for the case of the VVER- 440 MA vector with the gadolinium content of 2 and 2.5 wt% (Fig. 5). Sooner or later the k-inf in the three cases became smaller than the reference case. However, the cycle length with gadolinium content of 2.5 and 3 wt% was almost the same with the reference case while that with gadolinium content of 2 wt% was somewhat shorter. Consequently, TRAN VINH THANH et al. 23 reducing the gadolinium content to 3 wt% and boron concentration to 300 ppm is recommended when coating with 10 wt% of MA to the UGD pellets. The transmutation of MA isotopes when coating with 10 wt% of MAs and reducing the gadolinium content to 3 wt% and boron concentration to 300 ppm is given in Table III. Comparing Tables III and II, it is shown that the difference in the transmutation rate of MA isotopes between homogeneous and heterogeneous loadings was relatively small. However, the transmutation mass in the case of heterogeneous loading was slightly higher than that with homogeneous loading, in particular for the case of VVER- 440 MA vector. Table III also signify that the highest total MA transmutation mass and efficiency was again achieved for the case of loading the VVER-1000 MA vector as compared to the two other cases. Fig. 5. The k-inf of the LEU fuel assembly versus burnup when coating a layer of MAs to the UGD pins and reducing GD to 2 wt% (upper), 2.5 wt% (middle) and 3 wt% (lower) STUDY ON TRANSMUTATION EFFICIENCY OF THE VVER-1000 FUEL ASSEMBLY 24 Table III. Transmutation capability in case of heterogeneous loading of 10 wt% MA Isotope VVER-440 MA vector PWR-1000 MA vector VVER-1000 MA vector Initial amount (g) Mass reduced after 306 days Initial amount (g) Mass reduced after 306 days Initial amount (g) Mass reduced after 306 days (g) (%) (g) (%) (g) (%) 237 Np 896.79 150.40 16.77 766.06 118.35 15.45 0.00 __ __ 241 Am 580.05 238.34 41.09 877.12 317.87 36.24 1536.83 494.08 32.15 243 Am 269.51 51.13 18.97 157.98 24.03 15.21 241.56 34.39 14.23 244 Cm 81.53 -41.27 -50.62 29.87 -25.87 -86.61 50.12 -36.42 -72.67 245 Cm 4.79 -7.81 -142.27 1.65 -3.44 -208.80 4.17 -4.85 -116.46 Total 1832.67 391.79 21.38 1832.67 430.93 23.51 1832.67 484.39 26.43 IV. CONCLUSIONS In this study, the efficiency of MA transmutation as burnable poison in the VVER-1000 LEU fuel assembly was examined using the SRAC code for the MA homogeneous and heterogeneous loading parterns with different vectors of MAs recycled from the spent fuel of VVER-440, PWR-1000, and VVER-1000 reactors. The gadolinium content and the boron concentration were reduced correspondingly to compensate the negative reactivity insertion by MA loading. The results show that, with 10 wt% of MAs loading, 2.5-3.0 wt% of gadolinium content and 400-350 ppm of boron concentration were recommended for homogeneous mixing MAs in the UGD pins while 3 wt% of gadolinium content and 300 ppm of boron concentration were recommended for heterogeneous loading of MAs in the UGD pins. It was also found that 237 Np, 241 Am, and 243 Am could be significantly transmuted with a transmutation rate as high as ~40% for 241 Am. With this transmutation capability, burning MAs in the VVER-1000 fuel assembly could contribute to a significant reduction of radiotoxicity level of the radioactive waste since more than 90% of the radiotoxidity of MAs from long time storage spent fuel (more than hundred years) come from 241 Am (half-life of 432 years). Furthermore, the case of loading the VVER-1000 MA vector is highly recommended as it could lead to the highest 241 Am transmutation mass as well as the highest total MA transmutation mass and efficiency. In addition, it was shown that the MAs loading in combination with the reduction in gadolinium and boron concentration could help facilitate the excess reactivity control at the beginning of the fuel cycle without significant effect on the cycle length. Moreover, the MA coating approach could increase slightly the MA burning efficiency when comparing with homogeneous MA mixing model because of the self shielding effect on MAs, especially for the VVER-440 MA vector. Besides, the results indicate that, although loading of different MA vectors slightly affected the fuel cycle length, loading the MA vectors with lower amount of 237 Np and higher amount of 241 Am could help significantly reduce the excess reactivity at the beginning of the cycle. TRAN VINH THANH et al. 25 Further investigation on transmutation of MAs and radiotoxicity reduction at a full core level and MOX core of the VVER-1000 reactor is being planned. ACKNOWLEDGMENTS This research is funded by Vietnam National Foundation for Science and Technology Development (NAFOSTED) under grant number 103.99-2018.32. REFERENCES [1]. OECD/NEA, Minor Actinide Burning in Thermal Reactors, Nuclear Energy Agency, NEA No. 6997, 2013. [2]. Robert Jubin, Spent Fuel Reprocessing, Introduction to Nuclear Chemistry and Fuel Cycle Separations Course, Consortium for Risk Evaluation with Stakeholder Participation, ourse/, 2008. [3]. C.H.M. Broeders, E. Kiefhaber, H.W. Wiese, Burning transuranium isotopes in thermal and fast reactors, Nuclear Engineering and Design 202, 157–172, 2000. [4]. Z. Perkó, J. L. Kloosterman, S. Fehér, Minor actinide transmutation in GFR600, Nuclear Technology, Vol. 177, No. 1, pp. 83-97, January 2012. [5]. Timothée Kooyman, Laurent Buiron, Gérald Rimpault, A comparison of curium, neptunium and americium transmutation feasibility, Annals of Nuclear Energy 112 (2018) 748–758, https://doi.org/10.1016/j.anucene.2017.09.041. [6]. H. N. Tran, Y. Kato, New 237Np burning strategy in a supercritical CO2-cooled fast reactor core attaining zero burnup reactivity loss, Nuclear Science Engineering 159, 83-93, 2008. [7]. H. N. Tran, Y. Kato, P. H. Liem, V. K. Hoang, and S. M. T. Hoang, “Minor actinide transmutation in supercritical-CO2-cooled and sodium-cooled fast reactors with low burnup reactivity swings,” Nuclear Technology, vol. 205, no. 11, pp. 1460–1473, 2019. [8]. Vladimir Sebian, Vladimir Necas, Petr Darilek, Transmutation of spent fuel in reactor VVER- 440, Journal of Electrical Engineering, Vol. 52, No. 9-10, 299-302, 2001. [9]. B. R. Bergelson, A. S. Gerasimov, G. V. Tikhomirov, Transmutation of actinide in power reactors, Radiation Protection Dosimetry, Vol. 116, No. 1–4, pp. 675–678, 2005, doi:10.1093/rpd/nci249. [10]. Eugene Shwageraus, Pavel Hejzlar, Mujid S. Kazimi, A combined nonfertile and UO2 PWR fuel assembly for actinide waste minimization, Nuclear Technology, Vol. 149, March 2005. [11]. T. A. Taiwo, T. K. Kim, J. A. Stillman, R. N. Hill, M. Salvatores, P. J. Finck, Assessment of a heterogeneous PWR assembly for plutonium and minor actinide recycle, Nuclear Technology, Vol. 155, July 2006. [12]. Michael A. Pope, R. Sonat Sen, Abderrafi M. Ougouag, Gilles Youinou, Brian Boer, Neutronic analysis of the burning of transuranics in fully ceramic micro-encapsulated tri-isotropic particle- fuel in a PWR, Nuclear Engineering and Design 252, 215– 225, 2012, [13]. Bin Liu, Kai Wang, Jing Tu, Fang Liu, Liming Huang, Wenchao Hua, Transmutation of minor actinide in the pressurized water reactors, Annals of Nuclear Energy 64 (2014) 86–92, [14]. Bin Liu, Rendong Jia, Ran Han, Xuefeng Lyu, Jinsheng Han, Wenqiang Li, Minor actinide transmutation characteristics in AP1000, Annals of Nuclear Energy 115 (2018) 116–125, https://doi.org/10.1016/j.anucene.2018.01.031. [15]. Wenchao Hu, Bin Liu, Xiaoping Ouyang, Jing Tu, Fang Liu, Liming Huang, Juan Fu, Haiyan Meng, Minor actinide transmutation on PWR burnable poison rods, Annals of Nuclear Energy 77 (2015) 74–82, STUDY ON TRANSMUTATION EFFICIENCY OF THE VVER-1000 FUEL ASSEMBLY 26 [16]. Wenchao Hu, Jianping Jing, Jinsheng Bi, Chuanqi Zhao, Bin Liu, Xiaoping Ouyang, Minor actinide transmutation on pressurized water reactor burnable poison rods, Annals of Nuclear Energy 110 (2017) 222–229, [17]. V. T. Tran et al., Study on Transmutation of Minor Actinides as Burnable Poison in VVER- 1000 Fuel, Science and Technology of Nuclear Installations, Volume 2019, Article ID 5769147, 2019. [18]. OECD/NEA, A VVER-1000 LEU and MOX Assembly Computational Benchmark, Nuclear Energy Agency, NEA/NSC/DOC 10, 2002. [19]. OECD/NEA, A VVER-1000 LEU and MOX Assembly Computational Benchmark, Nuclear Energy Agency, NEA/NSC/DOC 10, 2002. [20]. A. Kotchetkov, I. Krivitskiy, N. Rabotnov, A. Tsiboulia, S. Iougai, Calculation and experimental studies on minor actinide reactor transmutation, Proceedings of Fifth OECD/NEA Information Exchange Meeting on Actinide and Fission Product Partitioning and Transmutation, pp. 289-303, Mol, Belgium, 25-27 November 1998. [21]. K. Okumura, T. Kugo, K. Kaneko, and K. Tsuchihashi, SRAC2006: A Comprehensive Neutronics Calculation Code System, JAEA- Data/Code 2007-004, 2007.
File đính kèm:
study_on_transmutation_efficiency_of_the_vver_1000_fuel_asse.pdf