Truyền thông không dây qua bề mặt phản xạ thông minh
Trong hệ thống truyền thông không dây hiện nay, phương tiện truyền
tin được coi là đối tượng hoạt động ngẫu nhiên giữa máy phát và bộ
thu, điều này làm giảm chất lượng tín hiệu do các yếu tố tự phát của
sóng vô tuyến. Bên cạnh đó, mạng di động tốc độ cao thế hệ thứ 5
(5G) cũng như các thách thức của mạng 6G trong tương lai đòi hỏi
phải có các công nghệ hỗ trợ hiệu quả. Để có thể khắc phục các tác
động tiêu cực của sóng lan truyền, một số công trình được đề xuất
nhằm điều khiển kênh không dây theo hướng tối ưu hóa. Nhóm tác
giả sẽ tiến hành khảo sát các nghiên cứu về công nghệ bề mặt thông
minh giúp kiểm soát sóng vô tuyến, góp phần nâng cao hiệu năng
chung của hệ thống truyền tin. Bài viết cung cấp các thông tin về cấu
tạo, nguyên lý hoạt động, khả năng ứng dụng, tính ưu việt của bề mặt
phản xạ thụ động có thể tái cấu hình, một số mô hình ước lượng
kênh, đồng thời đề xuất các hướng phát triển cho các hệ thống vô
tuyến thông minh tích hợp công nghệ mới nổi này. Kết quả của bài
báo có thể được sử dụng làm nền tảng kiến thức, tài liệu tham khảo
trong học tập, nghiên cứu và giảng dạy thuộc lĩnh vực công nghệ
thông tin và truyền thông
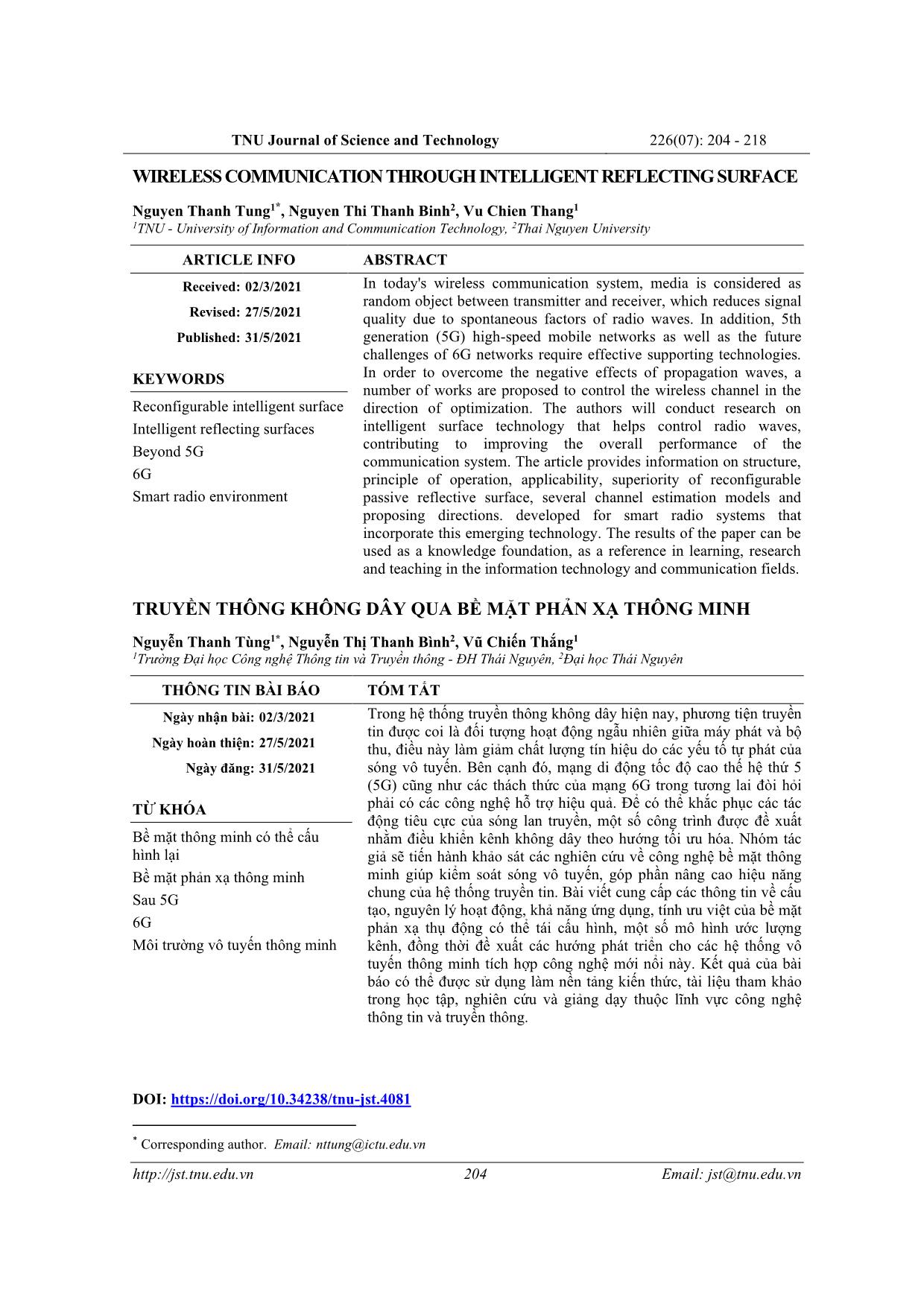
Trang 1
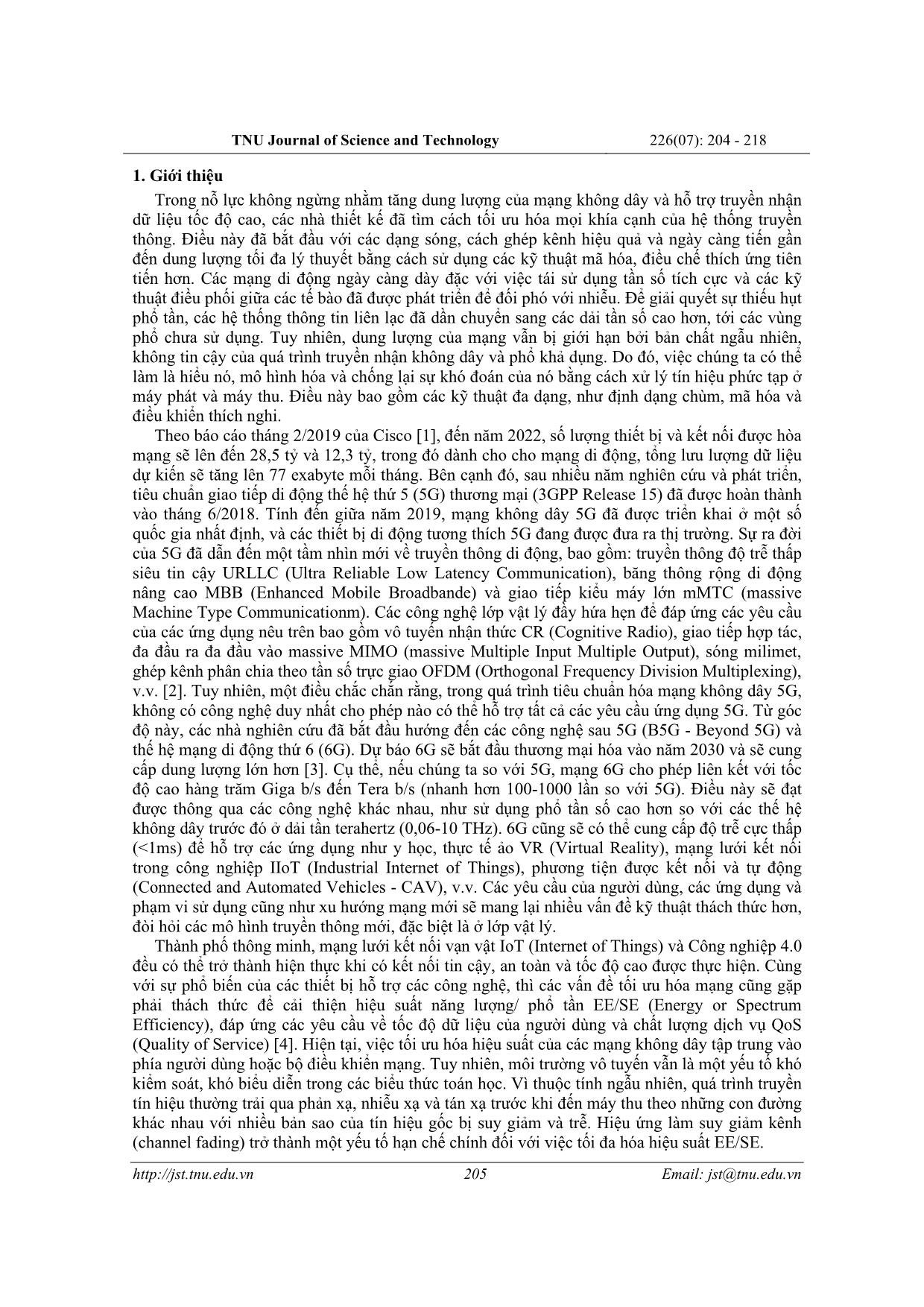
Trang 2
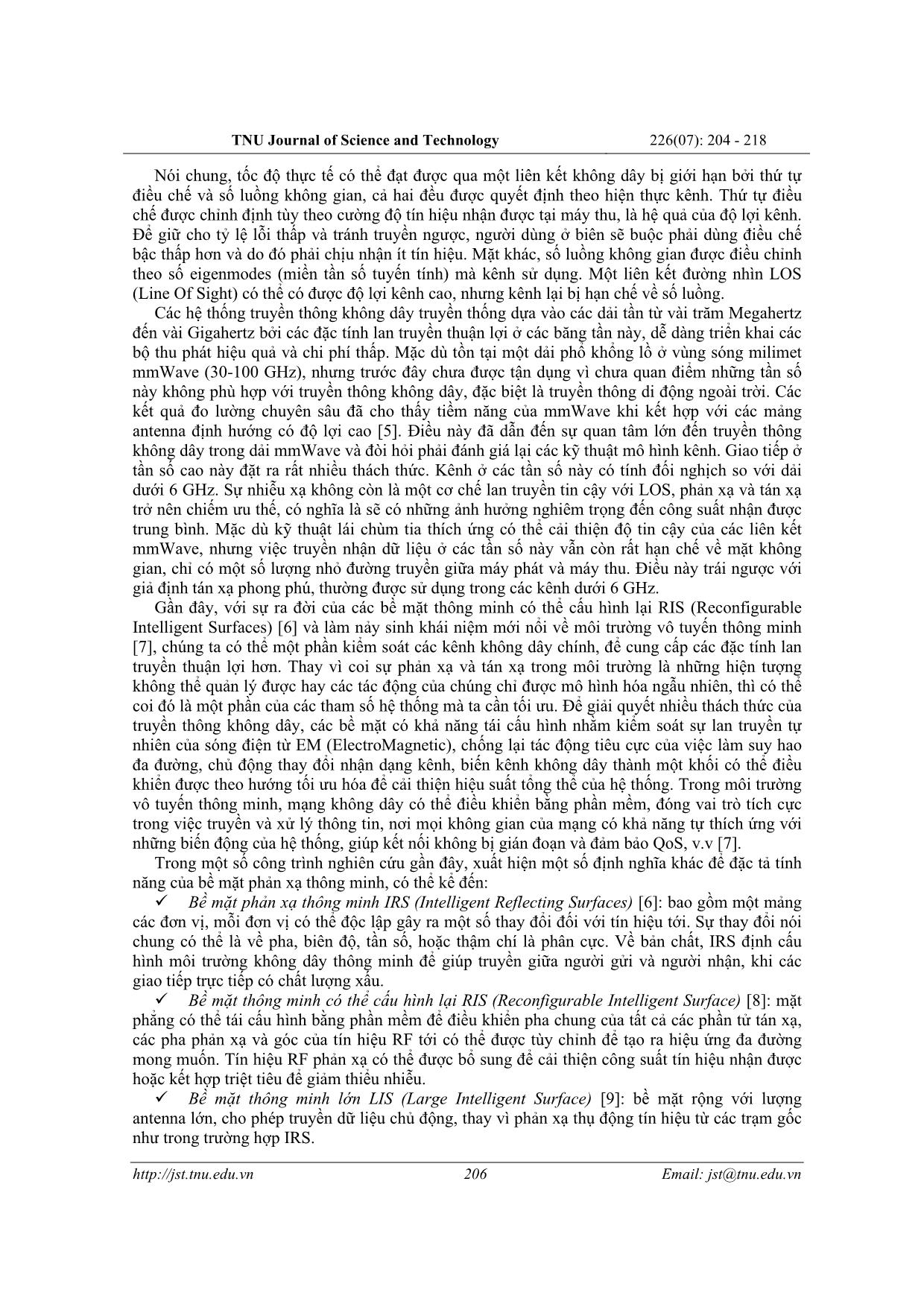
Trang 3
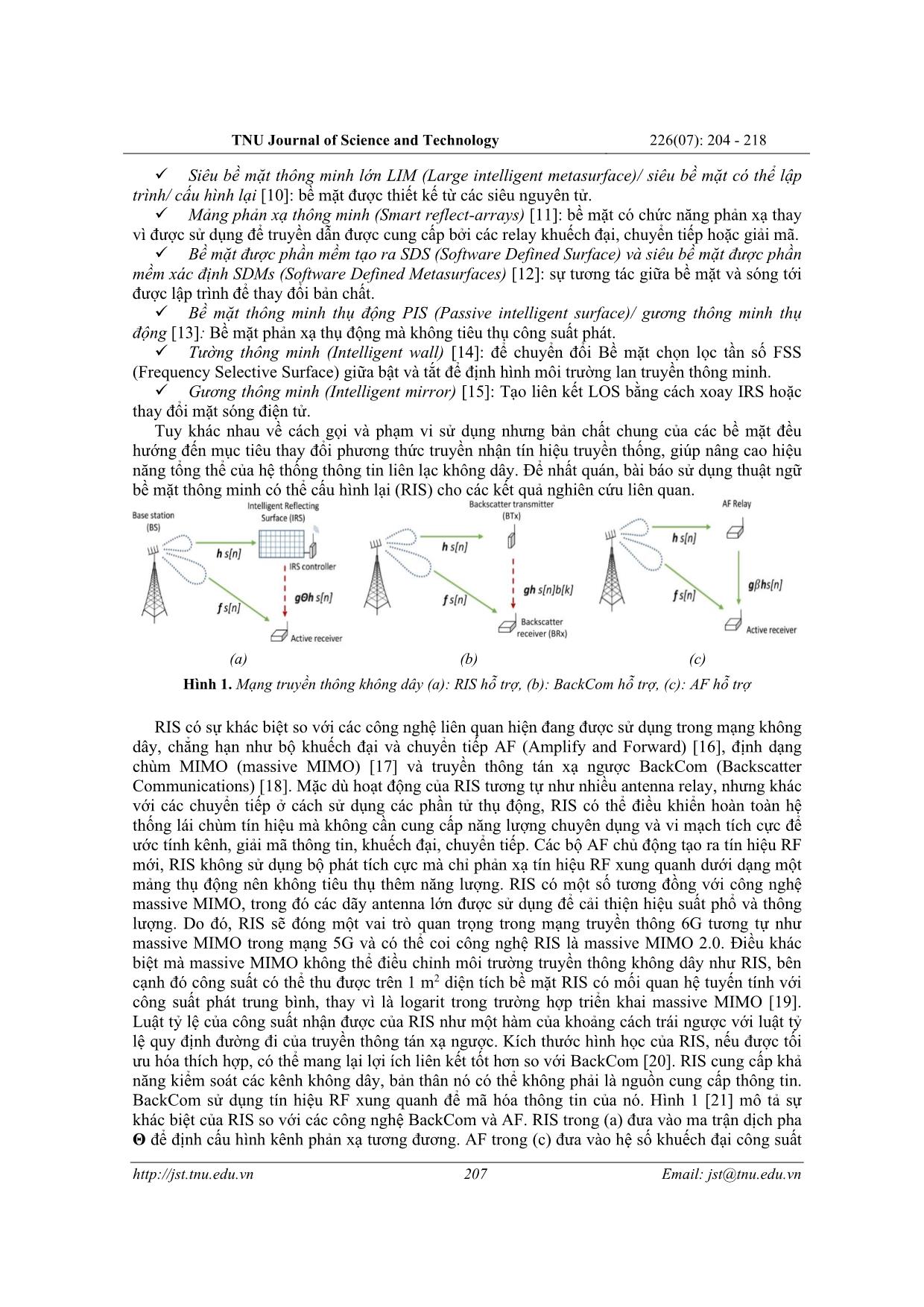
Trang 4
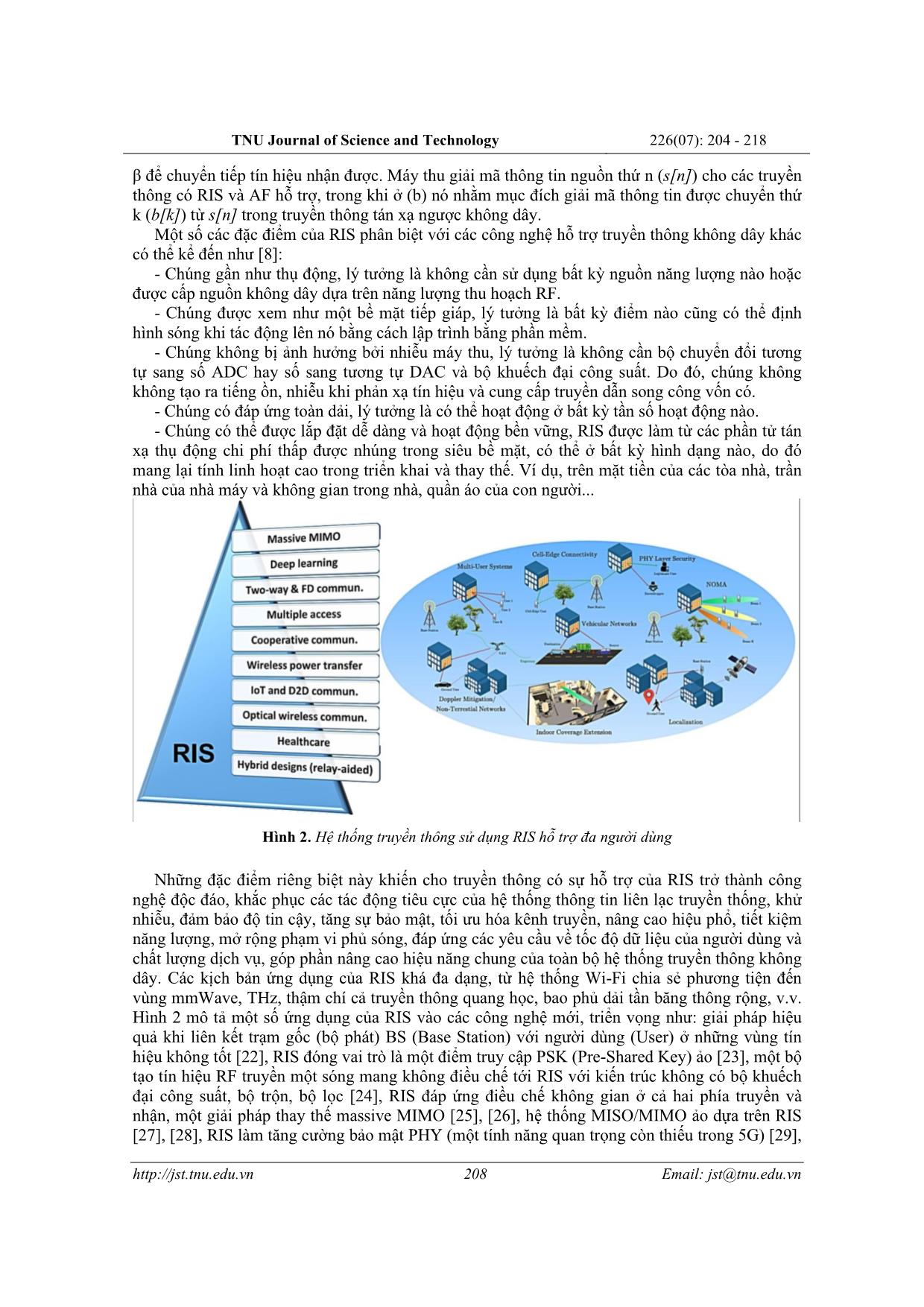
Trang 5
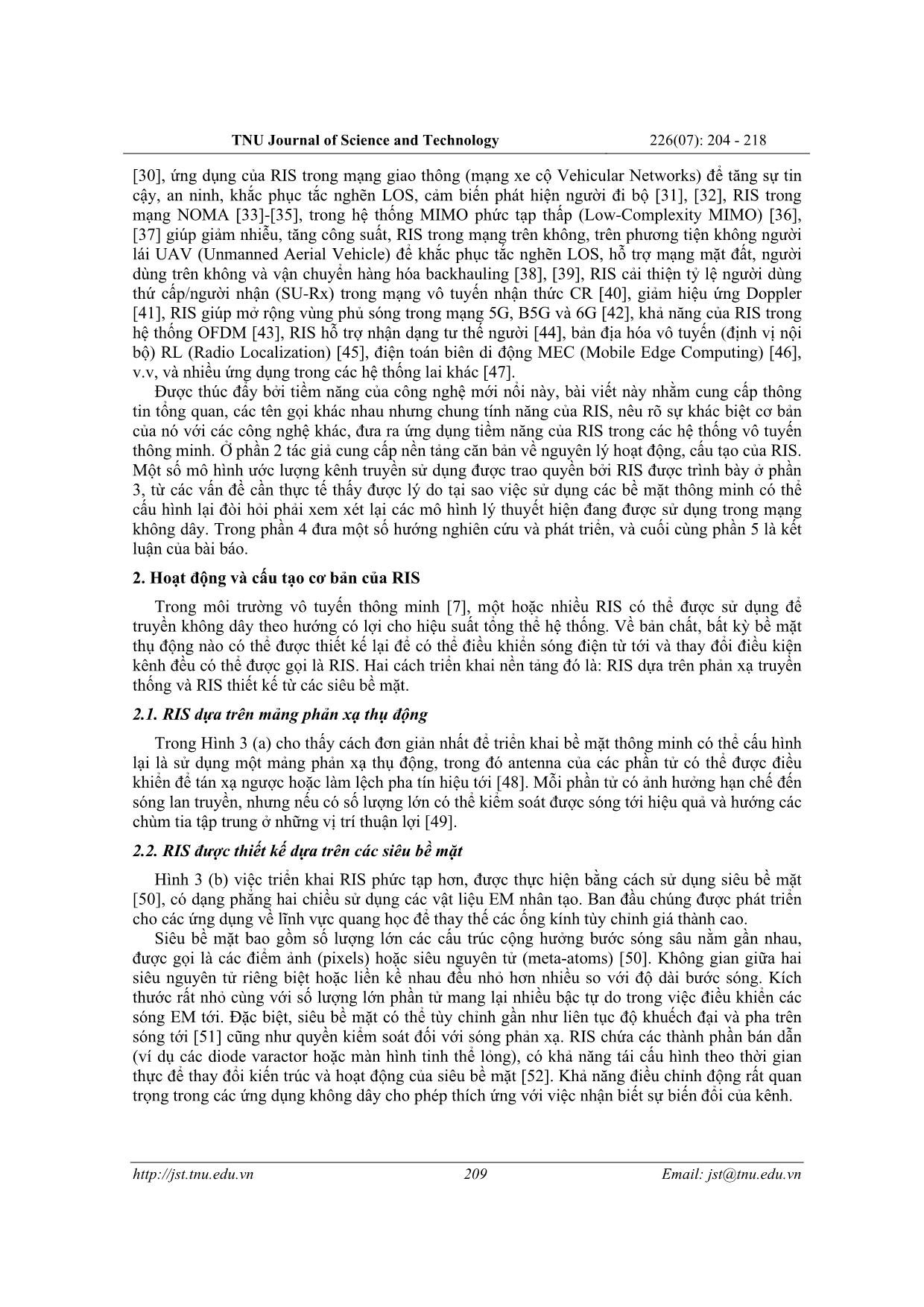
Trang 6
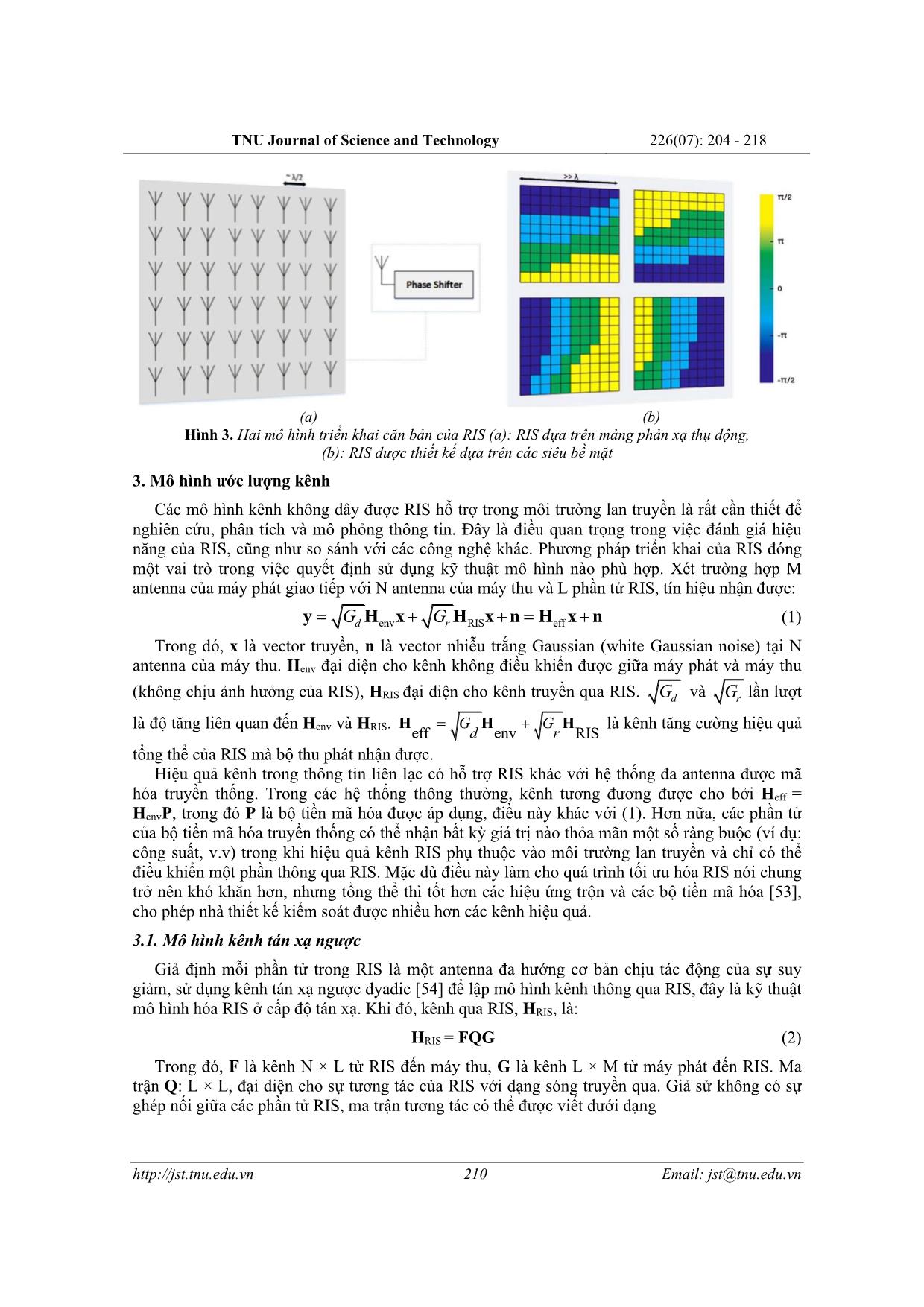
Trang 7
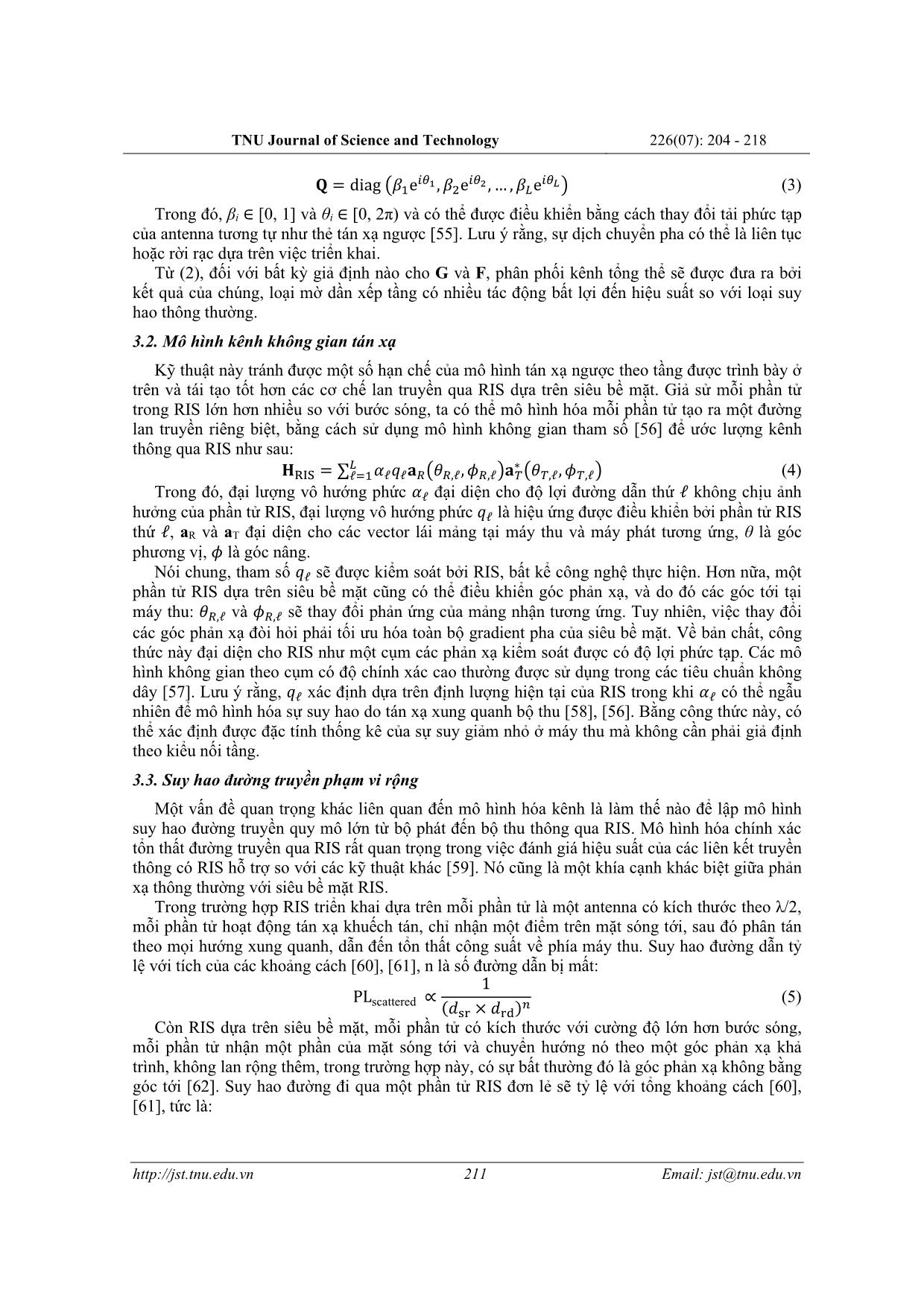
Trang 8
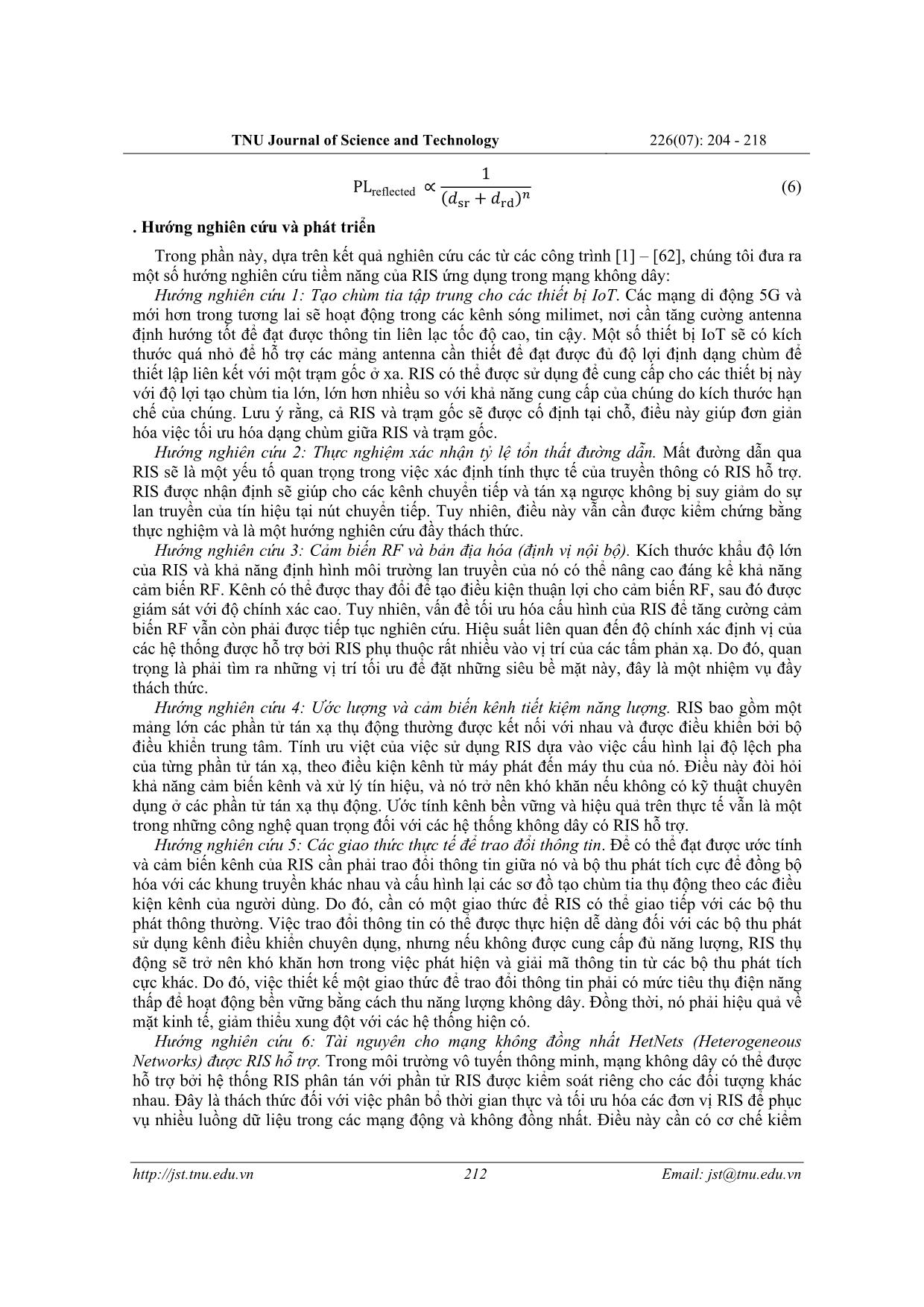
Trang 9
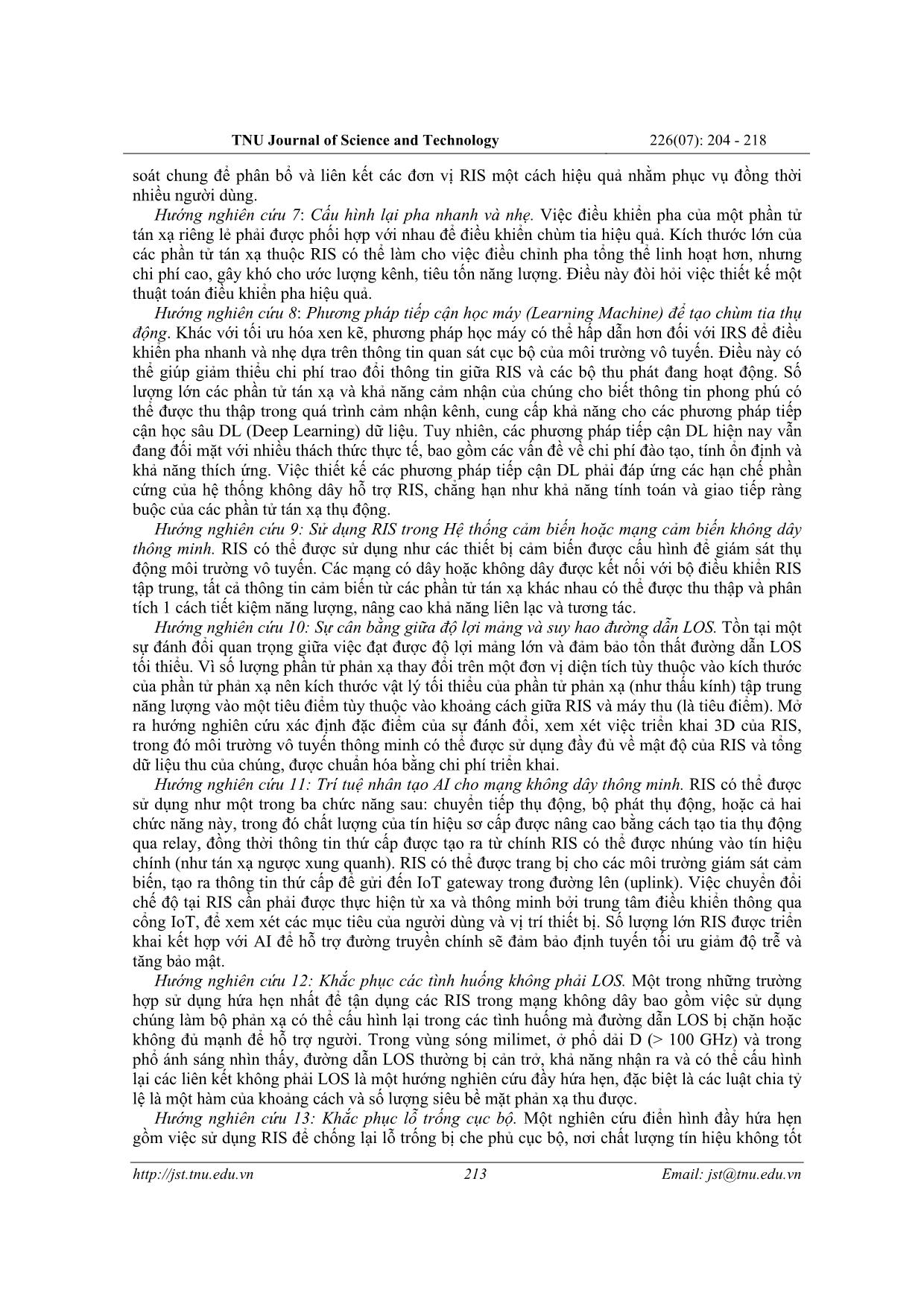
Trang 10
Tải về để xem bản đầy đủ
Tóm tắt nội dung tài liệu: Truyền thông không dây qua bề mặt phản xạ thông minh
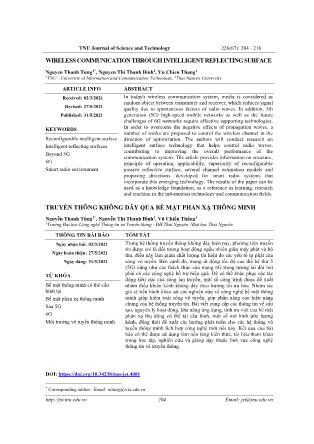
khám phá. Hướng nghiên cứu 25: Tích hợp RIS với mạng di động 5G, B5G, 6G. RIS là một trong những công nghệ lớp vật lý mang tính cách mạng và tiềm năng tạo ra mô hình truyền thông mới đáp ứng các yêu cầu của mạng 5G và B5G, 6G trong tương lai. Hệ thống lai này nâng cao hiệu quả quang phổ, cung cấp dịch vụ truyền thông không dây tin cậy, loại bỏ các thành phần nhiễu, cải thiện dung lượng mạng và hiệu suất của người dùng, đặc biệt đối với các môi trường mật độ người dùng cao như sân bay hay sân vận động. Do đó, đây là một hướng nghiên cứu thú vị, thông qua việc tính toán các ràng buộc và mục tiêu thích hợp khi thiết kế các hệ thống. Hướng nghiên cứu 26: Tích hợp RIS với các công nghệ mới. Sự kết hợp (hệ thống lai) của RIS với các công nghệ hiện đại và mới nổi, như tế bào nhỏ, MIMO lớn, truyền thông sóng milimet, truyền thông ánh sáng khả kiến VLC, giao tiếp terahertz, quang học không gian trống, truyền thông hỗ trợ máy bay không người lái (UAV), thu hoạch năng lượng EH, điện toán edge di động (MEC) và edge caching, truyền thông dưới nước, mạng mặt đất - vệ tinh v.v, là một hướng nghiên cứu đầy hứa hẹn và chưa được khám phá đầy đủ. 215 Email: jst@tnu.edu.vn TNU Journal of Science and Technology 226(07): 204 - 218 5. Kết luận Bài báo đã trình bày một cuộc khảo sát về vai trò của bề mặt phản xạ thông minh có thể cấu hình lại được RIS (Reconfigurable Intelligent Surfaces) đối với các mạng truyền thông không dây, giúp khắc phục các tác động tiêu cực của hệ thống thông tin liên lạc truyền thống, giảm nhiễu, đảm bảo độ tin cậy, tăng sự bảo mật, tối ưu hóa kênh truyền, nâng cao hiệu phổ, tiết kiệm năng lượng, mở rộng phạm vi phủ sóng, đáp ứng các yêu cầu về tốc độ dữ liệu của người dùng và chất lượng dịch vụ, góp phần nâng cao hiệu năng chung của toàn bộ hệ thống truyền thông. Đầu tiên, chúng tôi đã đưa ra một số tên gọi khác nhau của RIS về các bề mặt thụ động có thể điều khiển sóng điện từ tới và thay đổi điều kiện kênh, nêu lên các tính năng giúp phân biệt với các công nghệ tương đồng khác là bộ khuếch đại, chuyển tiếp AF (Amplify and Forward), định dạng chùm MIMO (massive MIMO) và truyền thông tán xạ ngược BackCom (Backscatter Communications). Tiếp đến, nhóm tác giả giới thiệu các kịch bản ứng dụng có thể được hưởng lợi từ khả năng cấu hình lại của nó trong các mạng không dây, các công nghệ mới nổi như: MIMO lớn, truyền thông sóng milimet, truyền thông ánh sáng khả kiến VLC, giao tiếp terahertz, truyền thông hỗ trợ máy bay không người lái (UAV), mạng 5G, B5G, 6G, v.v. Sau đó, bài viết cung cấp thông tin về hoạt động và cấu tạo cơ bản của RIS trong hai cách triển khai cơ bản đó là: RIS dựa trên phản xạ truyền thống và RIS thiết kế từ các siêu bề mặt. Ước lượng kênh không dây được RIS hỗ trợ trong bài báo giúp các nhà nghiên cứu, thiết kế phân tích mô phỏng thông tin và đánh giá hiệu năng của RIS. Cuối cùng, dựa trên các công trình, các ứng dụng có hiệu quả tích cực, các thách thức hiện tại, nhóm tác giả đề xuất các hướng nghiên cứu, phát triển tiềm năng trong tương lai, giúp cách mạng hóa công nghệ truyền thông không dây nói riêng và hệ thống thông tin liên lạc nói chung. TÀI LIỆU THAM KHẢO/ REFERENCES [1] “Cisco visual networking index: Global mobile data traffic forecast update, 2017–2022,” Feb. 2019. [Online]. Available: https://www.cisco.com/c/en/us/solutions/collateral/service-provider/visual- networking-index-vni/white-paper-c11-738429.pdf. [Accessed Feb. 14, 2021]. [2] M. Patzold, “It’s time to go big with 5G mobile radio,” IEEE Vehicular Technology Magazine, vol. 13, no. 4, pp. 4-10, 2018. [3] W. Saad, M. Bennis, and M. Chen, “A vision of 6G wireless systems: Applications, trends, technologies, and open research problems,” IEEE Network, vol. 34, no. 3, pp. 134 - 142, Oct. 2019. [4] X. Cao, L. Liu, Y. Cheng, and X. S. Shen, “Towards energy-efficient wireless networking in the big data era: A survey,” IEEE Commun. Surv. Tut., vol. 20, no. 1, pp. 303-332, Firstquarter 2018. [5] M. R. Akdeniz et al., “Millimeter wave channel modeling and cellular capacity evaluation,” IEEE J. Sel. Areas Commun., vol. 32, no. 6, pp. 1164-1179, Jun. 2014. [6] Q. Wu and R. Zhang, “Towards smart and reconfigurable environment: Intelligent reflecting surface aided wireless network,” IEEE Commun. Mag., vol. 58, no. 1, pp. 106-112, Jan. 2020. [7] M. D. Renzo et al., “Smart radio environments empowered by reconfigurable AI meta-surfaces: An idea whose time has come,” EURASIP J. Wireless Commun. Netw., vol. 2019, May 2019, Art. no. 129. [8] E. Basar, M. Di Renzo, J. de Rosny, M. Debbah, M.-S. Alouini, and R. Zhang, “Wireless communications through reconfigurable intelligent surfaces,” IEEE Access, vol. 7, pp. 116753- 116773, Aug. 2019. [9] E. Basar, “Transmission through large intelligent surfaces: A new frontier in wireless communications,” European Conference on Networks and Communications (EuCNC), 2019. [10] Z.-Q. He and X. Yuan, “Cascaded channel estimation for large intelligent metasurface assisted massive MIMO,” IEEE Wireless Communications Letters, vol. 9, pp. 210 - 214, Oct. 2019. [11] X. Tan, Z. Sun, D. Koutsonikolas, and J. M. Jornet, “Enabling indoor mobile millimeter-wave networks based on smart reflect-arrays,” IEEE Conference on Computer Communications (INFOCOM), 2018, pp. 270-278. [12] T. Ulversoy, “Software defined radio: Challenges and opportunities,” IEEE Communications Surveys & Tutorials, vol. 12, no. 4, pp. 531-550, 2010. [13] D. Mishra and H. Johansson, “Channel estimation and low-complexity beamforming design for passive intelligent surface assisted MISO wireless energy transfer,” IEEE International Conference on Acoustics, Speech and Signal Processing (ICASSP), 2019, pp. 4659-4663. 216 Email: jst@tnu.edu.vn TNU Journal of Science and Technology 226(07): 204 - 218 [14] L. Subrt and P. Pechac, “Controlling propagation environments using intelligent walls,” proc. European Conf. Antennas Propag. (EUCAP), Mar. 2012, pp. 26-30. [15] M. Najafi and R. Schober, “Intelligent reflecting surfaces for free space optical communications,” proc. IEEE GLOBECOM, Dec. 2019, pp. 1-7. [16] Z. Li et al., “Towards programming the radio environment with large arrays of inexpensive antennas,” Proc. USENIX Symp. Netw. Syst. Design Implement., Feb. 2019, pp. 285-299. [17] L. Sanguinetti, E. Bj¨ornson, and J. Hoydis, “Towards massive MIMO 2.0: Understanding spatial correlation, interference suppression, and pilot contamination,” IEEE Transactions on Communications, vol. 68, no. 1, pp. 232 - 257, 2019. [18] S. Y. Park and D. I. Kim, “Intelligent reflecting surface-aided phaseshift backscatter communication,” proc. Int. Conf. Ubiquit. Inf. Manag. Commun. (IMCOM), Jan. 2020, pp. 1-5. [19] S. Hu, F. Rusek, and O. Edfors, “The potential of using large antenna arrays on intelligent surfaces,” IEEE 85th Vehicular Technology Conference (VTC Spring), 2017, pp. 1-6. [20] H. Zheng, Z. Yang, G. Wang, R. He, and B. Ai, “Channel estimation for ambient backscatter communications with large intelligent surface,” Proc. IEEE Int. Conf. Wireless Commun. Signal Process. (WCSP), Oct. 2019, pp. 1-5. [21] S. Gong et al., “Towards Smart Wireless Communications via Intelligent Reflecting Surfaces: A Contemporary Survey,” IEEE Commun. Tuts. & Tuts., vol. 22, no. 4, pp. 2283-2314, June 2020. [22] C. Huang et al., “Reconfigurable intelligent surfaces for energy efficiency in wireless communication,” IEEE Trans. Wireless Commun., vol. 18, no. 8, pp. 4157-4170, 2019. [23] E. Basar, “Transmission through large intelligent surfaces: A new frontier in wireless communications,” EuCNC 2019, June 2019, pp. 18-21. [24] W. Tang et al., “Programmable metasurface-based RF chain-free 8PSK wireless transmitter,” Electron. Lett., vol. 55, no. 7, pp. 417-420, Apr. 2019. [25] E. Basar, “Reconfigurable Intelligent Surface-Based Index Modulation: A New Beyond MIMO Paradigm for 6G,” IEEE Trans. Commun., vol. 68, no. 5, pp. 3187-3196, 2020. [26] A. Canbilen, E. Basar, and S. Ikki, “Reconfigurable Intelligent Surface-Assisted Space Shift Keying,” IEEE Wireless Commun. Lett., vol. 9, no. 9, pp. 1495-1499, 2020. [27] A. Khaleel and E. Basar, “Reconfigurable Intelligent Surface-Empowered MIMO Systems,” IEEE Systems J. (to appear), July 2020. [28] W. Tang et al., “MIMO Transmission through Reconfigurable Intelligent Surface: System Design, Analysis, and Implementation,” IEEE J. Sel. Areas Commun., vol. 38, no. 11, pp. 2683-2699, 2020. [29] R. Zhang et al., “Secure Wireless Communication via Intelligent Reflecting Surface,” IEEE Wireless Commun. Lett., vol. 8, no. 5, pp. 1410-1414, 2019. [30] R. Schober et al., “Enabling Secure Wireless Communications via Intelligent Reflecting Surfaces,” GLOBECOM, 2019. [31] R. Schober et al., “Physical Layer Security in Vehicular Networks with Reconfigurable Intelligent Surfaces,” IEEE 91st Vehicular Technology Conference (VTC2020-Spring), Dec. 2019, pp. 25-28. [32] B. Massini et al., “The Use of Meta-Surfaces in Vehicular Networks,” J. Sens. Actuator Netw., vol. 9, Mar. 2019, doi: 10.3390/jsan9010015. [33] M. Fu et al., “Intelligent Reflecting Surface for Downlink Non-Orthogonal Multiple Access Networks,” GLOBECOM 2019, Dec. 2019. [34] Z. Ding and H. V. Poor, “Simple Design of IRS-NOMA Transmission,” IEEE Commun. Lett., vol. 24, no. 5, pp. 1119-1123, May 2020. [35] J. Zuo, Y. Liu, E. Basar, and O. A. Dobre, “Intelligent Reflecting Surface Enhanced Millimeter-Wave NOMA Systems,” IEEE Commun. Lett., vol. 24, no. 11, pp. 2632-2636, June 2020. [36] Z. Yigit, E. Basar, and I. Altunbas, “Low Complexity Adaptation for Reconfigurable Intelligent Surface-Based MIMO Systems,” IEEE Commun. Lett., vol. 24, no. 12, pp. 2946-2950, 2020. [37] L. Hanzo et al., “MIMO Assisted Networks Relying on Large Intelligent Surfaces: A Stochastic Geometry Model,” Oct. 2019. [Online]. Available: https://arxiv.org/abs/1910.00959. [Accessed Feb. 20, 2021]. [38] M. Bennis et al., “Reflections in the Sky: Millimeter Wave Communication with UAV-Carried Intelligent Reflectors,” IEEE Global Communications Conference (GLOBECOM), Feb. 2020, pp. 9-13. [39] S. Alfattani et al., “Aerial Platforms with Reconfigurable Smart Surfaces for 5G and Beyond,” in IEEE Communications Magazine, vol. 59, no. 1, pp. 96-102, 2021. 217 Email: jst@tnu.edu.vn TNU Journal of Science and Technology 226(07): 204 - 218 [40] E. Larsson et al., “Intelligent Reflecting Surface-Assisted Cognitive Radio System,” in IEEE Transactions on Communications, vol. 69, no. 1, pp. 675-687, 2021. [41] E. Basar, “Reconfigurable Intelligent Surfaces for Doppler Effect and Multipath Fading Mitigation”, Nov. 2019. [Online]. Available: https://arxiv.org/abs/1912.04080. [Accessed Jan. 15, 2021]. [42] I. Yildirim, A. Uyrus, and E. Basar, “Modeling and Analysis of Reconfigurable Int Applications in Future Wireless Networks,” IEEE Transactions on Communications, vol. 69, no. 2, pp. 1290-1301, 2021. [43] R. Zhang et al., “Intelligent Reflecting Surface Meets OFDM: Protocol Design and Rate Maximization,” June 2019. [Online]. Available: https://arxiv.org/abs/1906.09956. [Accessed Jan. 12, 2021]. [44] H. V. Poor et al., “Reconfigurable Intelligent Surfaces based RF Sensing: Design, Optimization, and Implementation,” IEEE Journal on Selected Areas in Communications, vol. 38, no. 11, pp. 2700- 2716, 2020. [45] H. Wymeersch et al., “Radio Localization and Mapping with Reconfigurable Intelligent Surfaces,” IEEE Vehicular Technology Magazine, vol. 15, no. 4, pp. 52-61, 2020. [46] S. Hua and Y. Shi, “Reconfigurable intelligent surface for green edge inference in machine learning,” proc. IEEE GLOBECOM Workshops, Dec. 2019, pp. 1-6. [47] Q. Wu et al., “Intelligent Reflecting Surface Aided Wireless Communications: A Tutorial,” EEE Transactions on Communications, vol. 69, no. 5, pp. 3313-3351, 2021. [48] S. V. Hum and J. Perruisseau-Carrier, “Reconfigurable reflectarrays and array lenses for dynamic antenna beam control: A review,” IEEE Trans. Antennas Propag., vol. 62, no. 1, pp. 183-198, Jan. 2014. [49] V. Arun and H. Balakrishnan, “RFocus: Practical beam- forming for small devices,” May 2019. [Online]. Available: [Accessed Jan. 10, 2021]. [50] H. Chen, A. J. Taylor, and N. Yu, “A review of metasurfaces: Physics and applications,” Rep. Progr. Phys., vol. 79, no. 7, Jun. 2016, Art. no. 076401. [51] C. Huang et al., “Holographic MIMO surfaces for 6G wireless networks: Opportunities, challenges, and trends,” IEEE Wireless Communications, vol. 27, no. 5, pp. 118-125, 2020. [52] Q. He, S. Sun, and L. Zhou, “Tunable/reconfigurable metasurfaces: Physics and applications,” Research, vol. 2019, pp. 1-16, Jul. 2019. [53] R. W. Heath and A. Lozano, Foundations of MIMO Communication, 1st ed. Cambridge, U.K.: Cambridge Univ. Press, Dec. 2018. [54] J. D. Griffin and G. D. Durgin, “Gains for RF tags using multiple antennas,” IEEE Trans. Antennas Propag., vol. 56, no. 2, pp. 563-570, Feb. 2008. [55] M. ElMossallamy, Z. Han, M. Pan, R. Jantti, K. Seddik, and G. Y. Li, “Noncoherent frequency shift keying for ambient backscatter over OFDM signals,” Proc. IEEE Int. Conf. Commun. (ICC), Shanghai, China, May 2019, pp. 1-6. [56] R. W. Heath, N. González-Prelcic, S. Rangan, W. Roh, and A. M. Sayeed, “An overview of signal processing techniques for millime- ter wave MIMO systems,” IEEE J. Sel. Topics Signal Process., vol. 10, no. 3, pp. 436-453, Apr. 2016. [57] P. Almers et al., “Survey of channel and radio propagation models for wireless MIMO systems,” EURASIP J. Wireless Commun. Netw., vol. 2007, Feb. 2007, Art. no. 019070. [58] O. E. Ayach, S. Rajagopal, S. Abu-Surra, Z. Pi, and R. W. Heath, “Spatially sparse precoding in millimeter wave MIMO systems,” IEEE Trans. Wireless Commun., vol. 13, no. 3, pp. 1499-1513, Mar. 2014. [59] E. Björnson, L. Sanguinetti, H. Wymeersch, J. Hoydis, and T. L. Marzetta, “Massive MIMO is a reality—What is next?: Fivepromising research directions for antenna arrays,” Digital SignalProcess., vol. 94, pp. 3-20, Nov. 2019. [60] T. Rappaport, Wireless Communications: Principles and Practice, 2nd ed. Upper Saddle River, NJ, USA: Prentice Hall PTR, 2001. [61] K. R. Schaubach, N. J. Davis, and T. S. Rappaport, “A ray tracingmethod for predicting path loss and delay spread in microcellular environments,” Vehicular Technology Society 42nd VTS Conference - Frontiers of Technology, May 1992, pp. 10-13. [62] A. Díaz-Rubio, V. S. Asadchy, A. Elsakka, and S. A. Tretyakov, “Fromthe generalized reflection law to the realization of perfect anomalousreflectors,” Sci. Adv., vol. 3, no. 8, Aug. 2017, Art. no. e1602714. 218 Email: jst@tnu.edu.vn
File đính kèm:
truyen_thong_khong_day_qua_be_mat_phan_xa_thong_minh.pdf