Radiation-crosslinked scaffolds from gelatin/CM-chitin and gelatin/CM-chitosan hydrogels for adipose-derived stem cell culture
Radiation technology has been providing a useful tool to modify polymers involving the
radiation crosslinking of hydrogels. In the present work, two kinds of hydrogels composed gelatin and
carboxymethyl chitosan (gelatin/CM-chitosan), and carboxymethyl chitin (gelatin/CM-chitin) were
prepared by the radiation crosslinking. The resulting hydrogels were freeze-dried, sterilized under
gamma-ray at a sterilization dose of 25 kGy, and characterized to be utilized as the scaffolds for
culturing adipose-derived stem cells (hADSCs). The physicochemical properties, biodegradability,
and cytotoxicity of these scaffolds were also investigated. The results indicated that both CM-chitosan
and CM-chitin much improved the swelling capacity, porosity, and pore size of the gelatin-derived
scaffolds. The swelling degree of gelatin/CM-chitosan and gelatin/CM-chitin scaffolds was about 7-9
g/g after 6 hrs immerging in PBS, and their porosity was about 70-73% with pore size ranging from
100 to 300 mm. The results also revealed that the compressive modulus of gelatin/CM-chitosan and
gelatin/CM-chitin was 45.6 and 66.4 kPa, respectively, which were suitable for soft tissue engineering.
Both scaffolds were enzymatically biodegradable by collagenase and non-cytotoxic for hADSCs with
an RGR of ~97%. Thus, the resultant scaffolds can be suitably utilized for culturing hADSC in
practice to regenerate soft tissues.
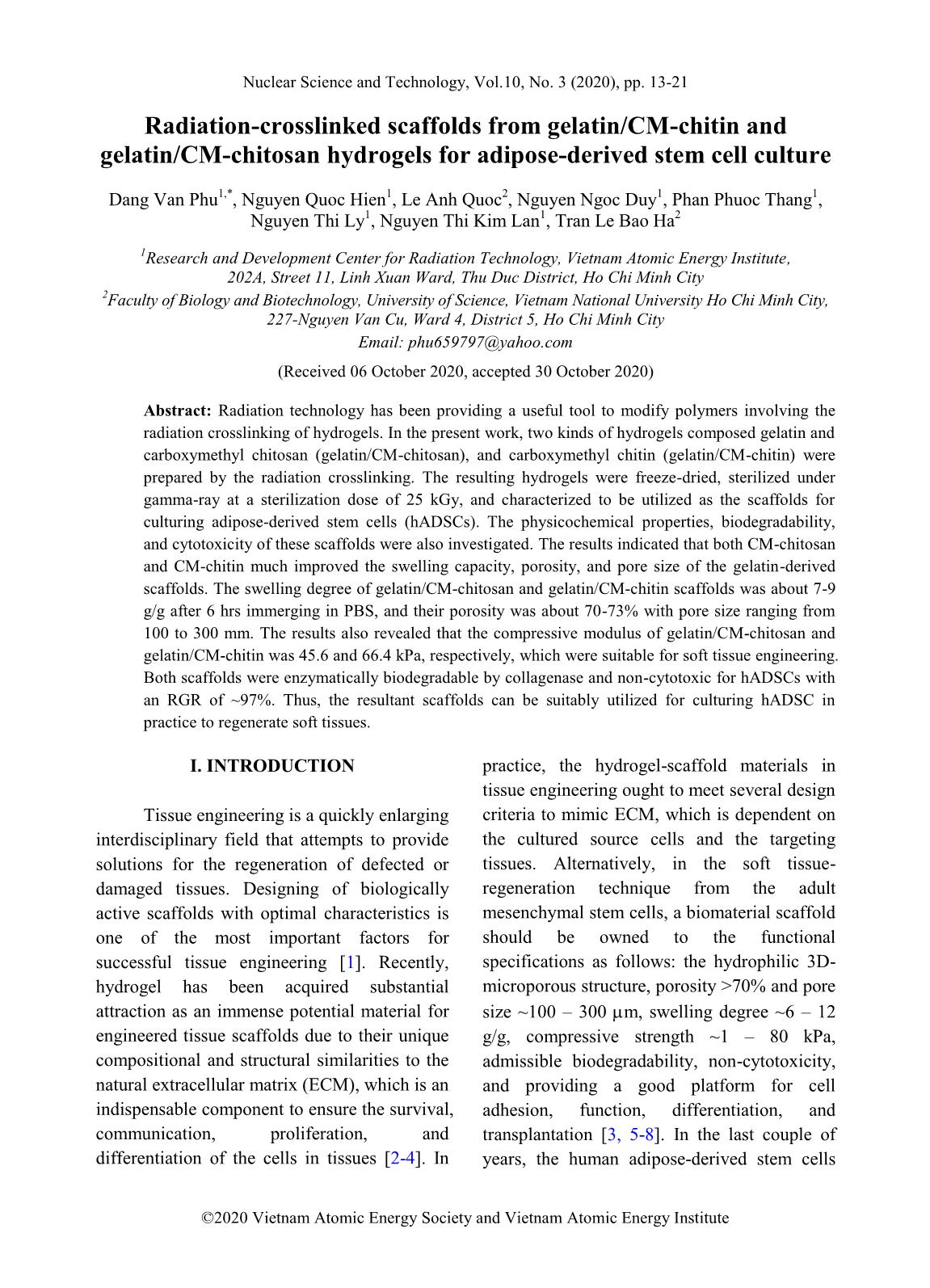
Trang 1
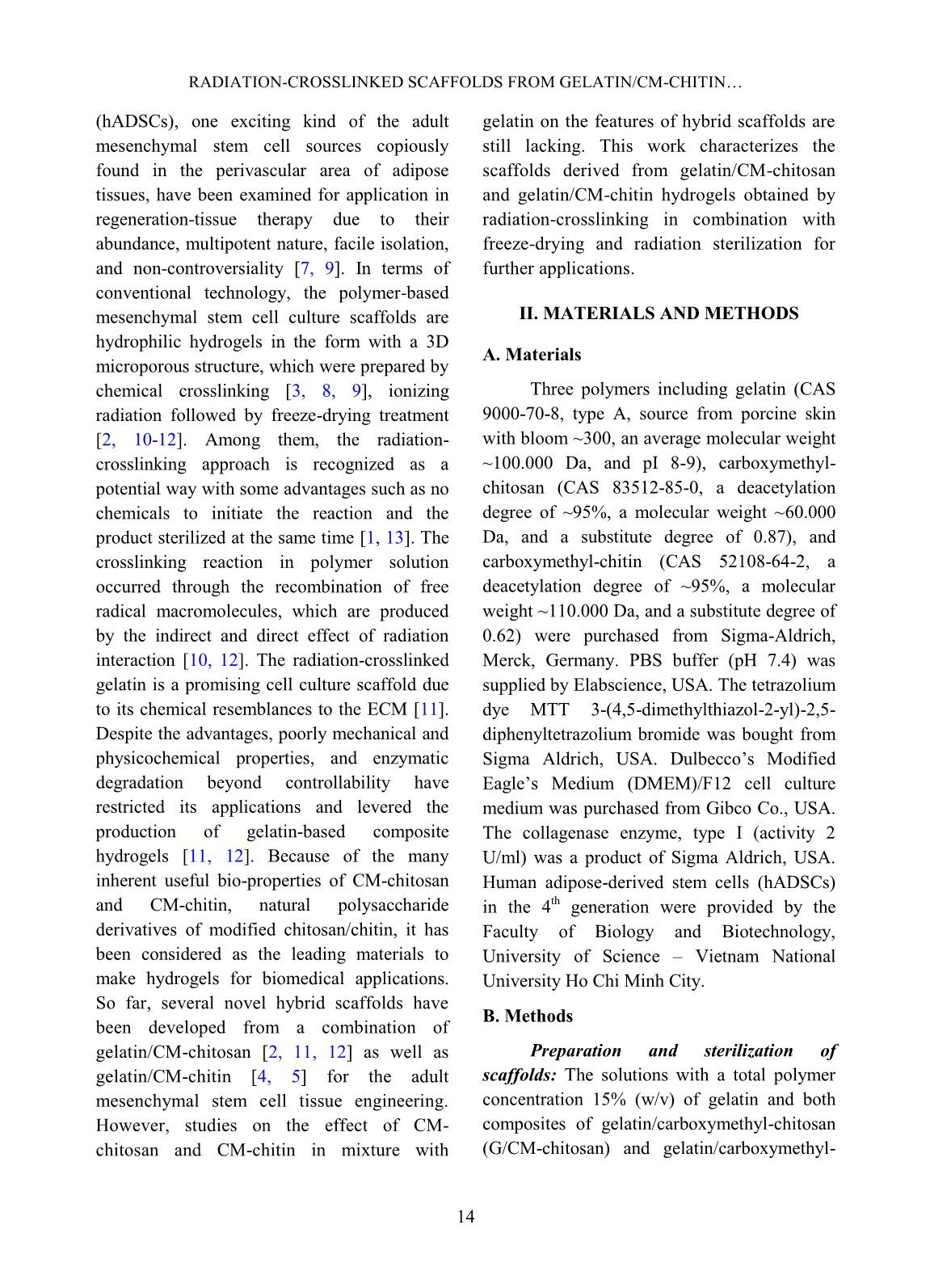
Trang 2
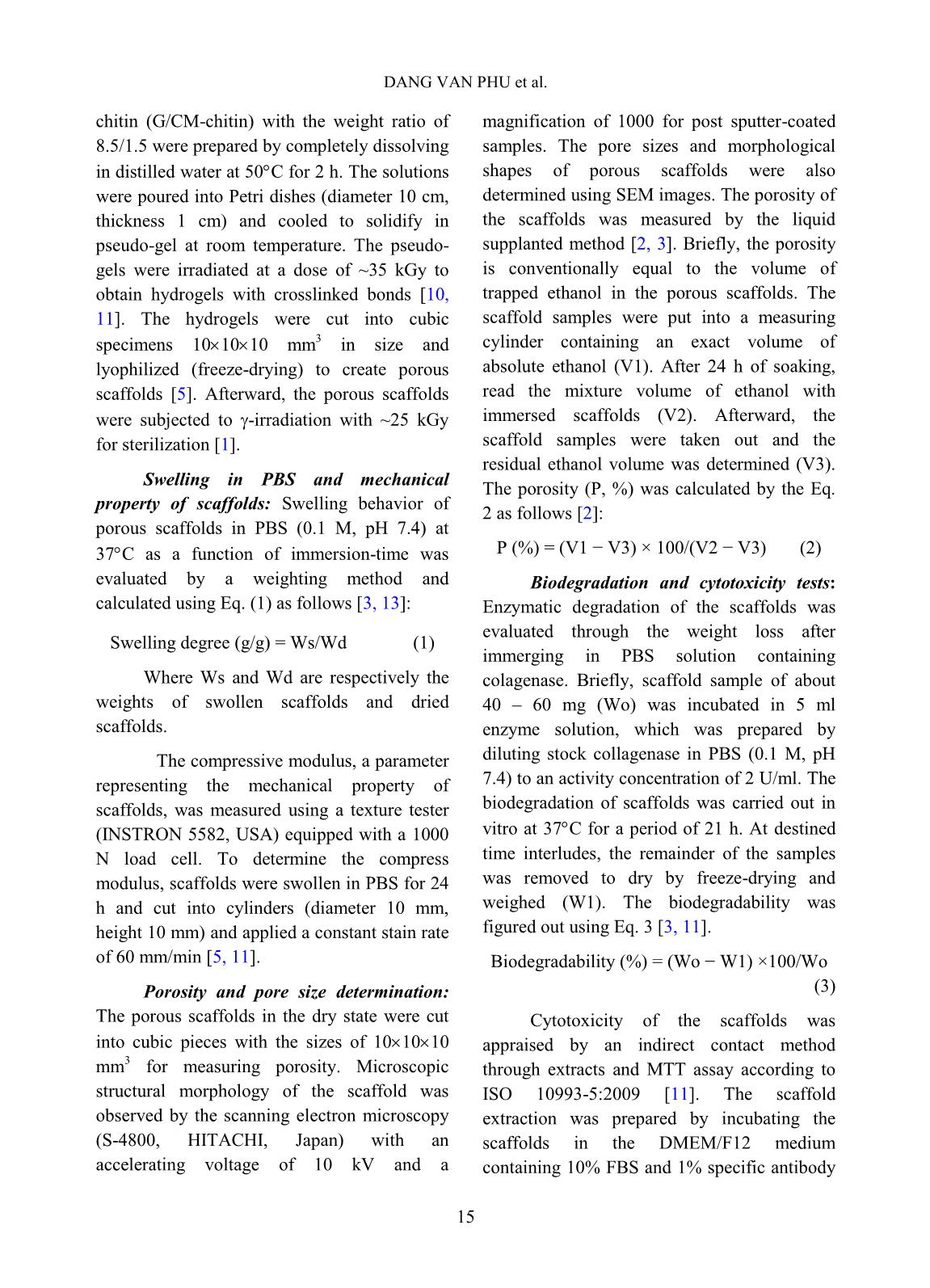
Trang 3
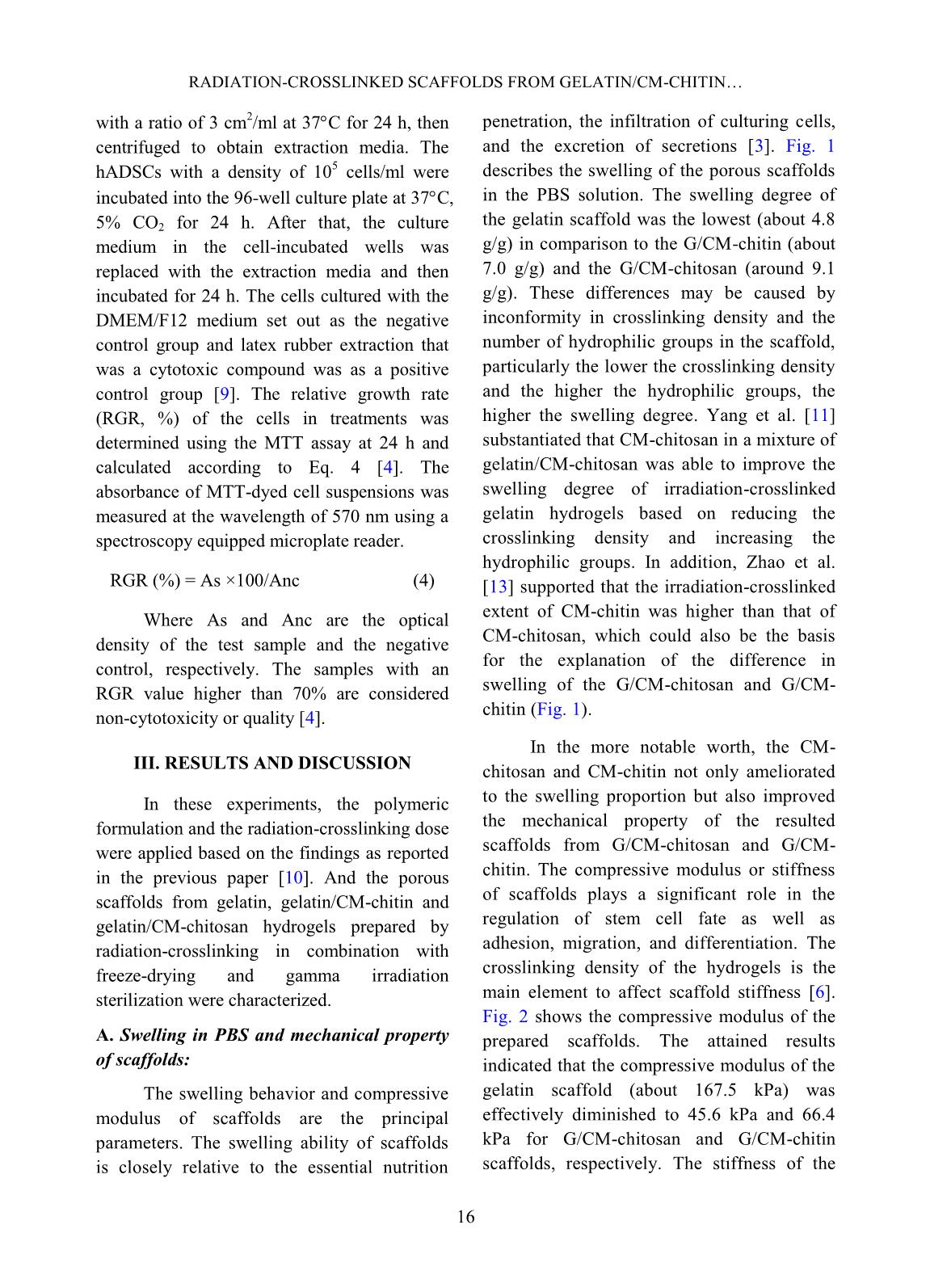
Trang 4
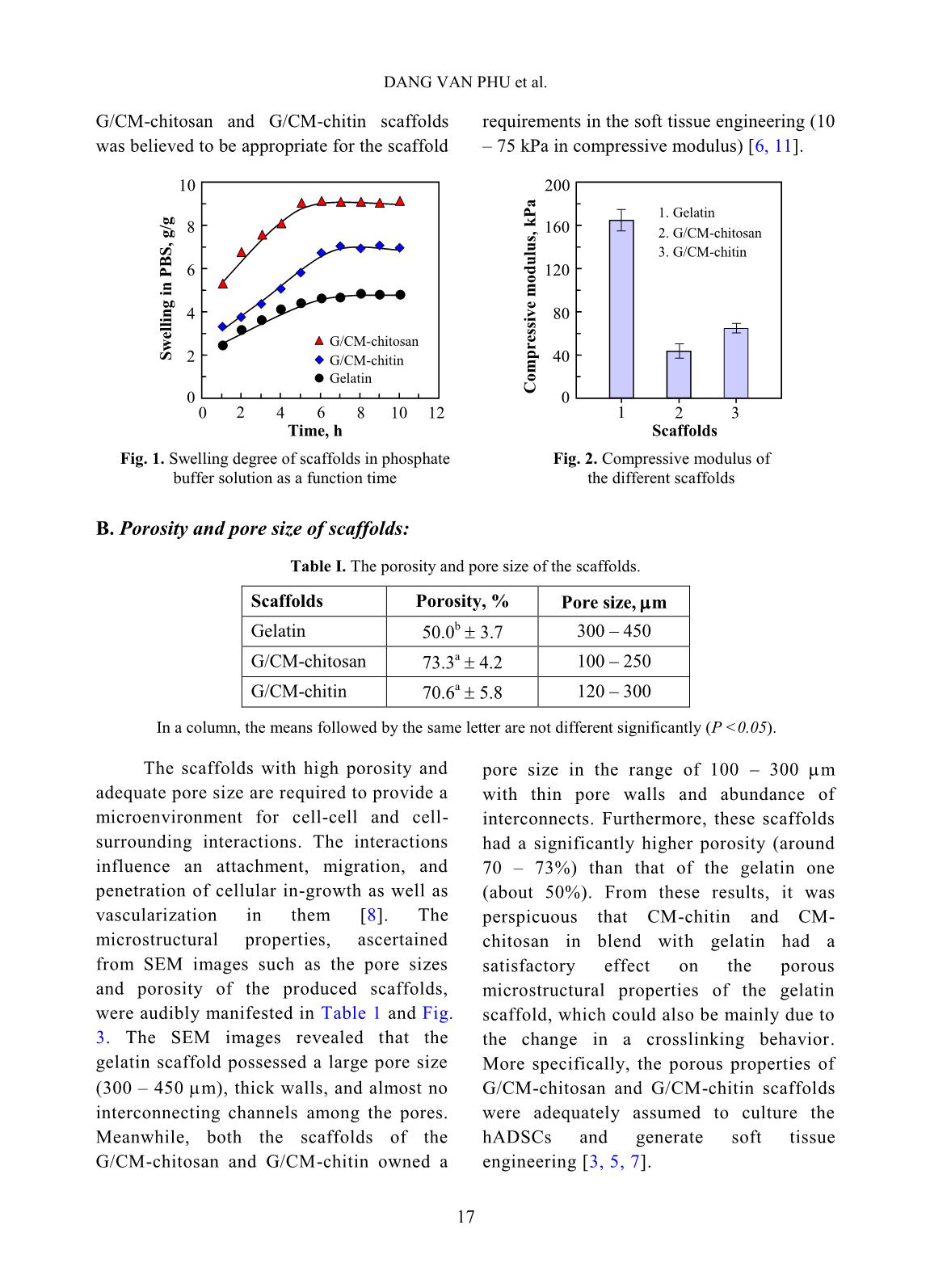
Trang 5
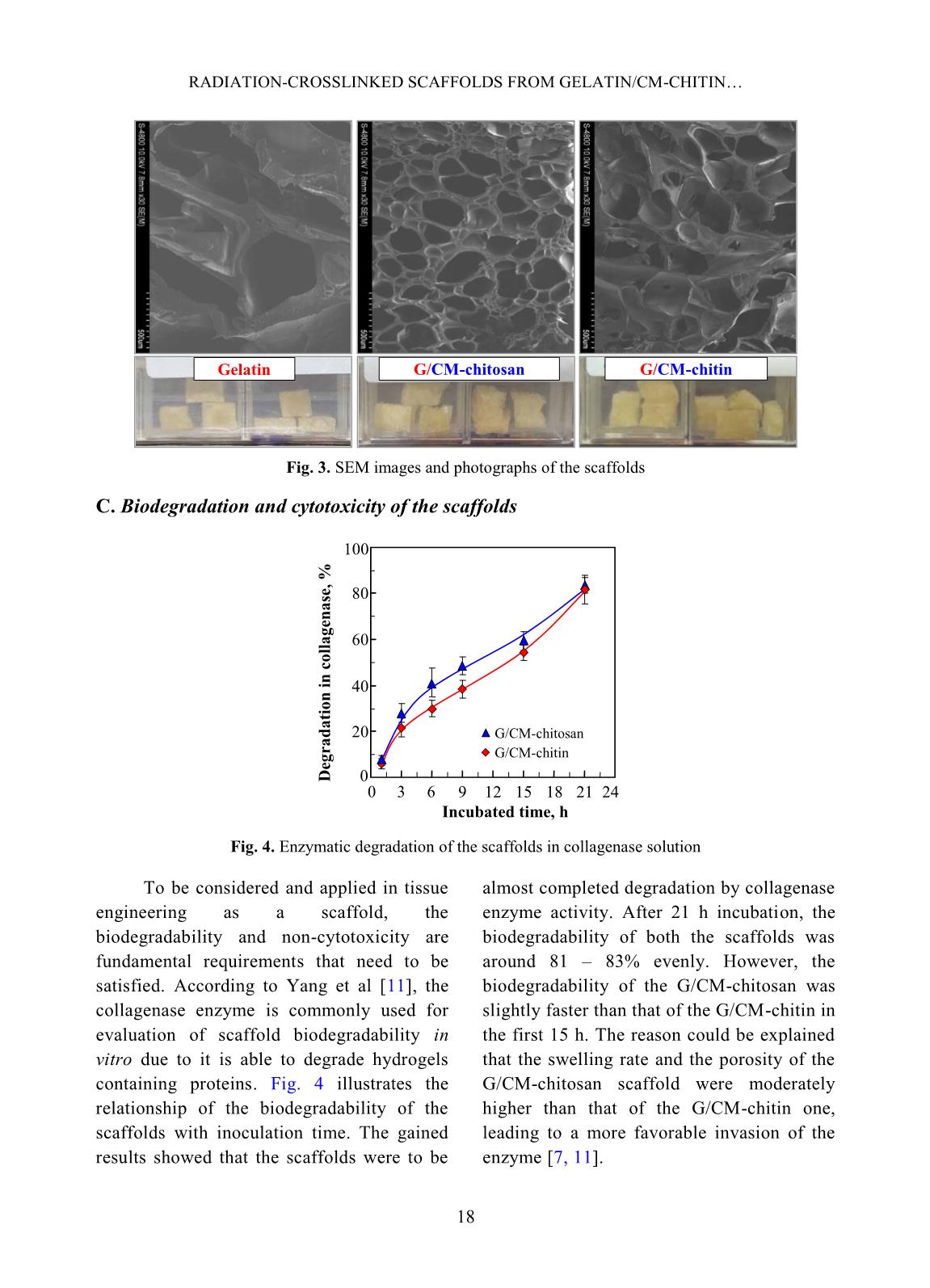
Trang 6
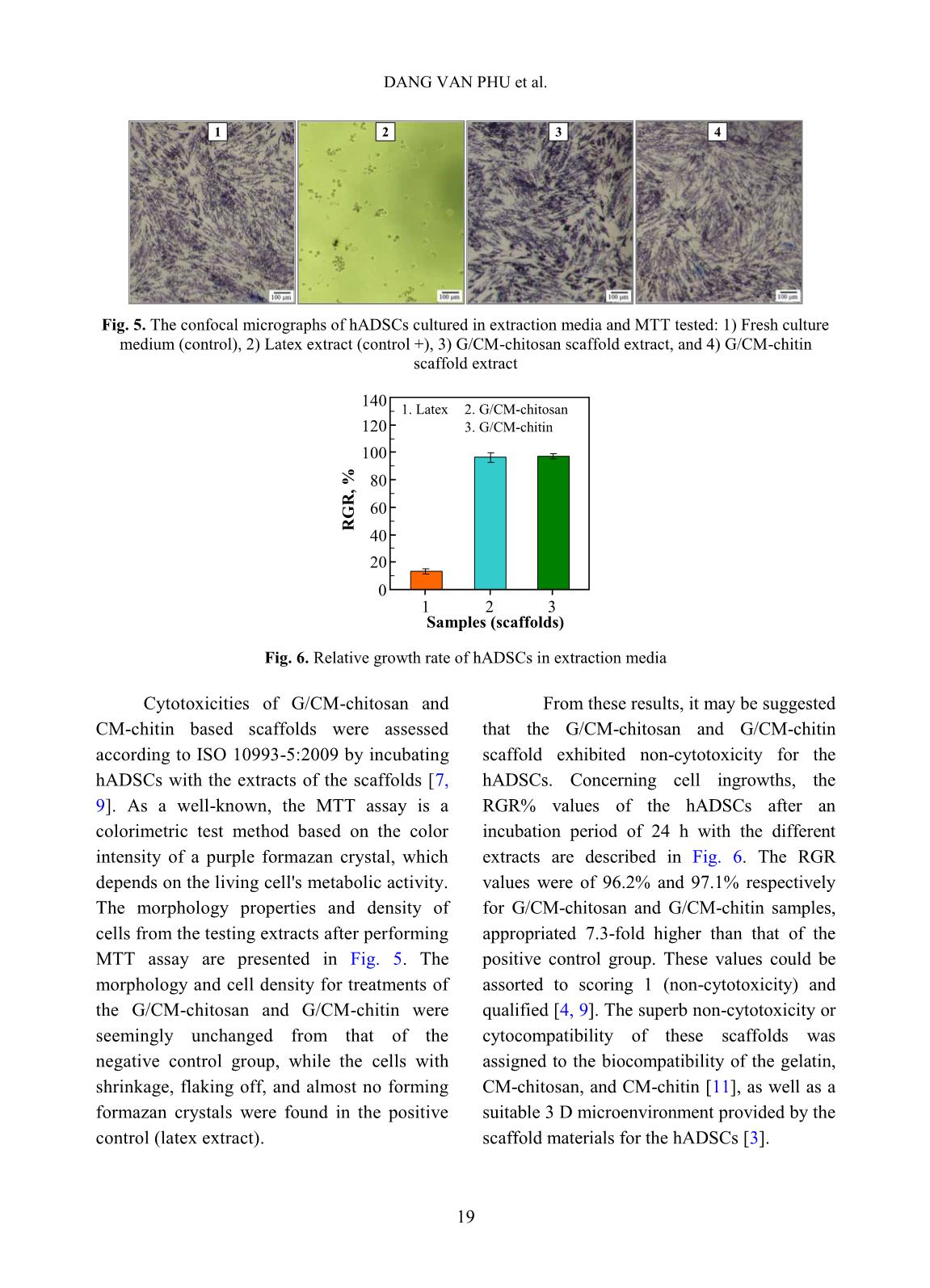
Trang 7
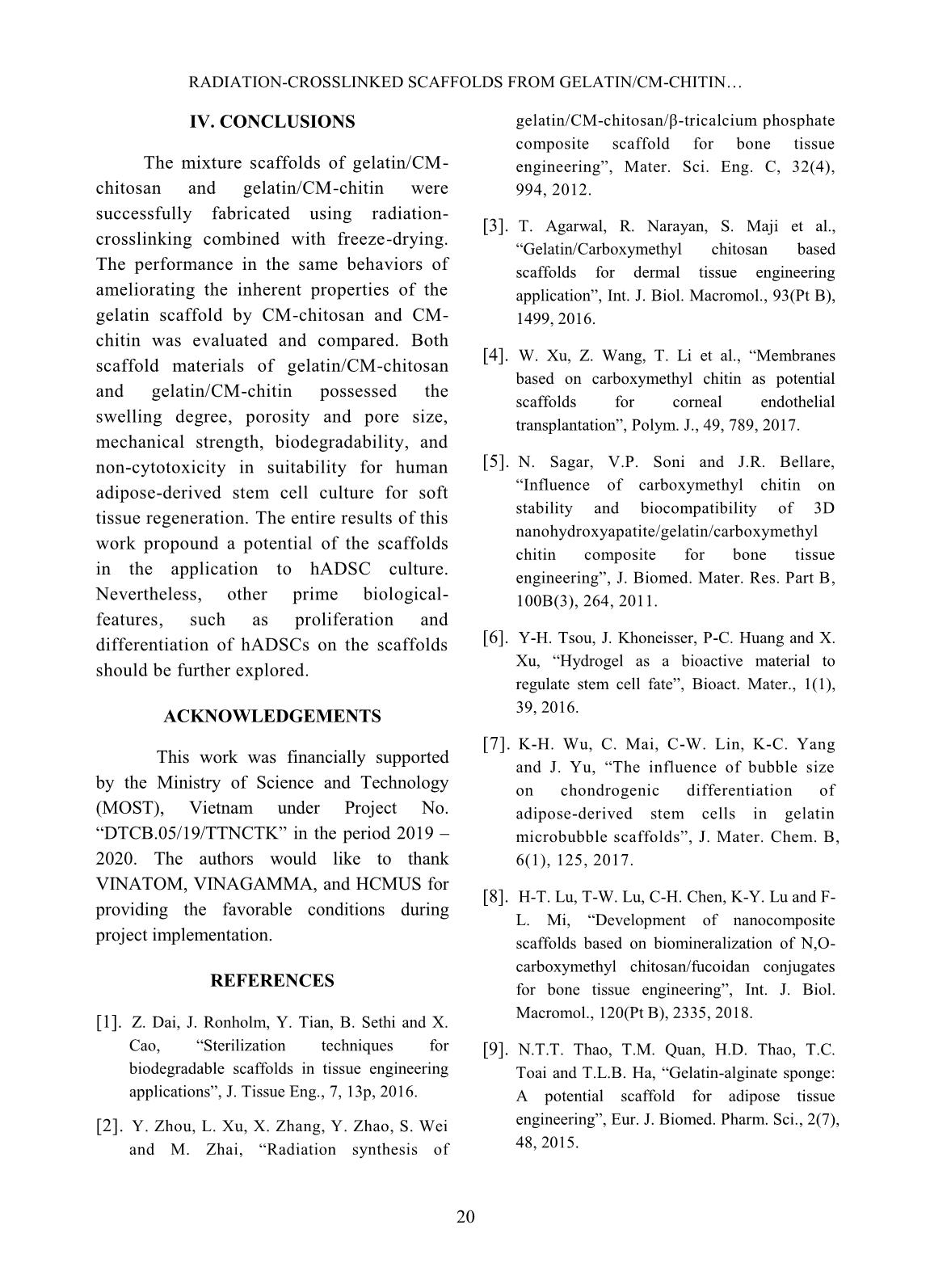
Trang 8
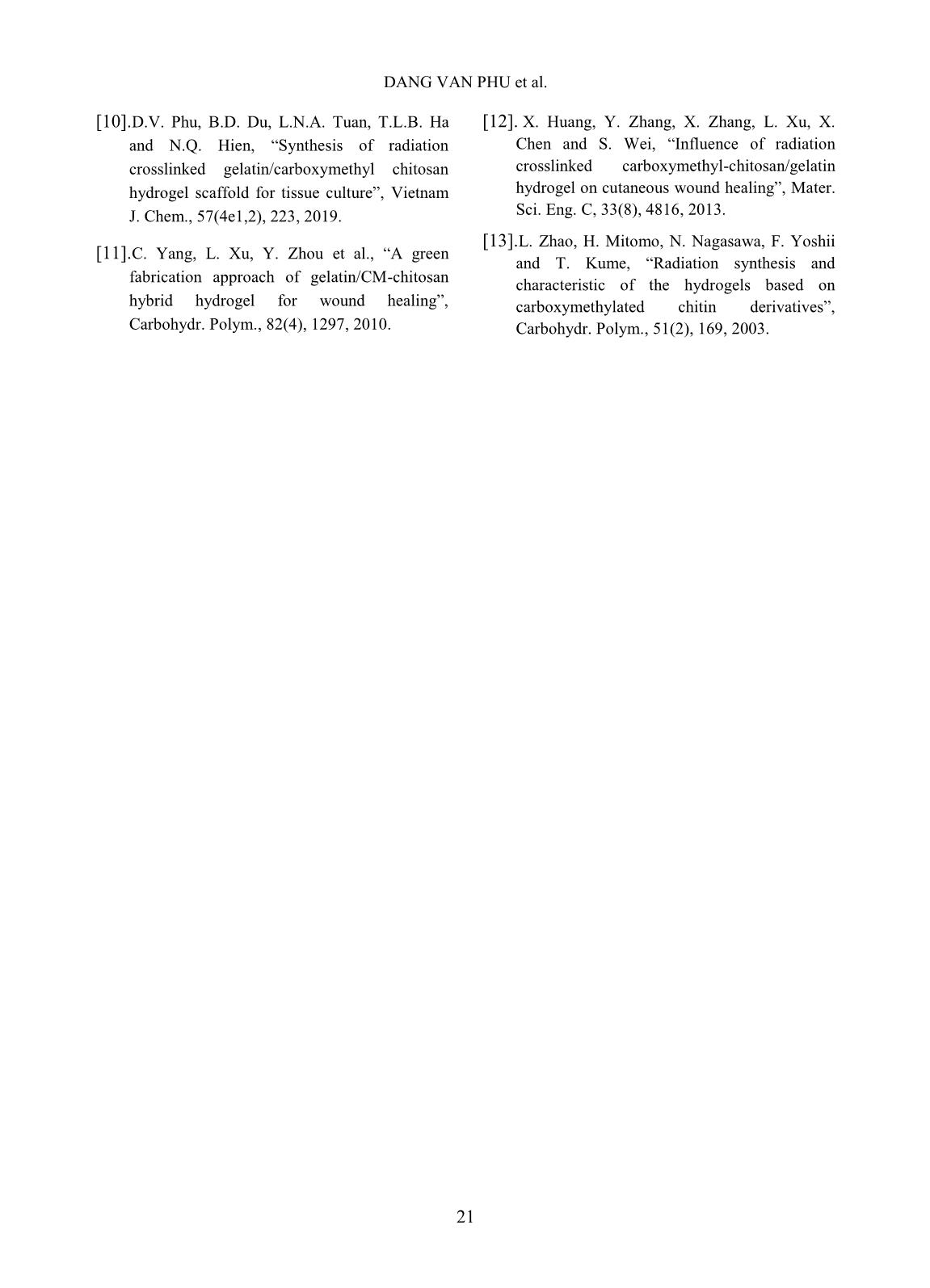
Trang 9
Tóm tắt nội dung tài liệu: Radiation-crosslinked scaffolds from gelatin/CM-chitin and gelatin/CM-chitosan hydrogels for adipose-derived stem cell culture
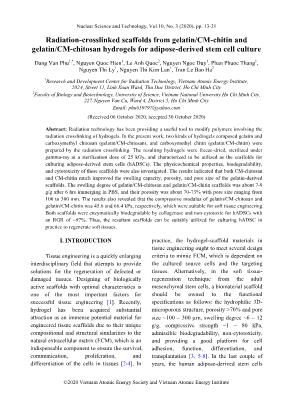
volume of ethanol with immersed scaffolds (V2). Afterward, the scaffold samples were taken out and the residual ethanol volume was determined (V3). The porosity (P, %) was calculated by the Eq. 2 as follows [2]: P (%) = (V1 − V3) × 100/(V2 − V3) (2) Biodegradation and cytotoxicity tests: Enzymatic degradation of the scaffolds was evaluated through the weight loss after immerging in PBS solution containing colagenase. Briefly, scaffold sample of about 40 – 60 mg (Wo) was incubated in 5 ml enzyme solution, which was prepared by diluting stock collagenase in PBS (0.1 M, pH 7.4) to an activity concentration of 2 U/ml. The biodegradation of scaffolds was carried out in vitro at 37C for a period of 21 h. At destined time interludes, the remainder of the samples was removed to dry by freeze-drying and weighed (W1). The biodegradability was figured out using Eq. 3 [3, 11]. Biodegradability (%) = (Wo − W1) ×100/Wo (3) Cytotoxicity of the scaffolds was appraised by an indirect contact method through extracts and MTT assay according to ISO 10993-5:2009 [11]. The scaffold extraction was prepared by incubating the scaffolds in the DMEM/F12 medium containing 10% FBS and 1% specific antibody RADIATION-CROSSLINKED SCAFFOLDS FROM GELATIN/CM-CHITIN 16 with a ratio of 3 cm 2 /ml at 37C for 24 h, then centrifuged to obtain extraction media. The hADSCs with a density of 10 5 cells/ml were incubated into the 96-well culture plate at 37C, 5% CO2 for 24 h. After that, the culture medium in the cell-incubated wells was replaced with the extraction media and then incubated for 24 h. The cells cultured with the DMEM/F12 medium set out as the negative control group and latex rubber extraction that was a cytotoxic compound was as a positive control group [9]. The relative growth rate (RGR, %) of the cells in treatments was determined using the MTT assay at 24 h and calculated according to Eq. 4 [4]. The absorbance of MTT-dyed cell suspensions was measured at the wavelength of 570 nm using a spectroscopy equipped microplate reader. RGR (%) = As ×100/Anc (4) Where As and Anc are the optical density of the test sample and the negative control, respectively. The samples with an RGR value higher than 70% are considered non-cytotoxicity or quality [4]. III. RESULTS AND DISCUSSION In these experiments, the polymeric formulation and the radiation-crosslinking dose were applied based on the findings as reported in the previous paper [10]. And the porous scaffolds from gelatin, gelatin/CM-chitin and gelatin/CM-chitosan hydrogels prepared by radiation-crosslinking in combination with freeze-drying and gamma irradiation sterilization were characterized. A. Swelling in PBS and mechanical property of scaffolds: The swelling behavior and compressive modulus of scaffolds are the principal parameters. The swelling ability of scaffolds is closely relative to the essential nutrition penetration, the infiltration of culturing cells, and the excretion of secretions [3]. Fig. 1 describes the swelling of the porous scaffolds in the PBS solution. The swelling degree of the gelatin scaffold was the lowest (about 4.8 g/g) in comparison to the G/CM-chitin (about 7.0 g/g) and the G/CM-chitosan (around 9.1 g/g). These differences may be caused by inconformity in crosslinking density and the number of hydrophilic groups in the scaffold, particularly the lower the crosslinking density and the higher the hydrophilic groups, the higher the swelling degree. Yang et al. [11] substantiated that CM-chitosan in a mixture of gelatin/CM-chitosan was able to improve the swelling degree of irradiation-crosslinked gelatin hydrogels based on reducing the crosslinking density and increasing the hydrophilic groups. In addition, Zhao et al. [13] supported that the irradiation-crosslinked extent of CM-chitin was higher than that of CM-chitosan, which could also be the basis for the explanation of the difference in swelling of the G/CM-chitosan and G/CM- chitin (Fig. 1). In the more notable worth, the CM- chitosan and CM-chitin not only ameliorated to the swelling proportion but also improved the mechanical property of the resulted scaffolds from G/CM-chitosan and G/CM- chitin. The compressive modulus or stiffness of scaffolds plays a significant role in the regulation of stem cell fate as well as adhesion, migration, and differentiation. The crosslinking density of the hydrogels is the main element to affect scaffold stiffness [6]. Fig. 2 shows the compressive modulus of the prepared scaffolds. The attained results indicated that the compressive modulus of the gelatin scaffold (about 167.5 kPa) was effectively diminished to 45.6 kPa and 66.4 kPa for G/CM-chitosan and G/CM-chitin scaffolds, respectively. The stiffness of the DANG VAN PHU et al. 17 G/CM-chitosan and G/CM-chitin scaffolds was believed to be appropriate for the scaffold requirements in the soft tissue engineering (10 – 75 kPa in compressive modulus) [6, 11]. B. Porosity and pore size of scaffolds: Table I. The porosity and pore size of the scaffolds. Scaffolds Porosity, % Pore size, m Gelatin 50.0 b 3.7 300 – 450 G/CM-chitosan 73.3 a 4.2 100 – 250 G/CM-chitin 70.6 a 5.8 120 – 300 In a column, the means followed by the same letter are not different significantly (P <0.05). The scaffolds with high porosity and adequate pore size are required to provide a microenvironment for cell-cell and cell- surrounding interactions. The interactions influence an attachment, migration, and penetration of cellular in-growth as well as vascularization in them [8]. The microstructural properties, ascertained from SEM images such as the pore sizes and porosity of the produced scaffolds, were audibly manifested in Table 1 and Fig. 3. The SEM images revealed that the gelatin scaffold possessed a large pore size (300 – 450 m), thick walls, and almost no interconnecting channels among the pores. Meanwhile, both the scaffolds of the G/CM-chitosan and G/CM-chitin owned a pore size in the range of 100 – 300 m with thin pore walls and abundance of interconnects. Furthermore, these scaffolds had a significantly higher porosity (around 70 – 73%) than that of the gelatin one (about 50%). From these results, it was perspicuous that CM-chitin and CM- chitosan in blend with gelatin had a satisfactory effect on the porous microstructural properties of the gelatin scaffold, which could also be mainly due to the change in a crosslinking behavior. More specifically, the porous properties of G/CM-chitosan and G/CM-chitin scaffolds were adequately assumed to culture the hADSCs and generate soft tissue engineering [3, 5, 7]. Time, h S w el li n g i n P B S , g /g 0 2 4 6 8 10 0 2 4 6 8 10 12 Gelatin G/CM-chitin G/CM-chitosan Scaffolds C o m p re ss iv e m o d u lu s, k P a 0 40 80 120 160 200 1 2 3 1. Gelatin 3. G/CM-chitin 2. G/CM-chitosan Fig. 1. Swelling degree of scaffolds in phosphate buffer solution as a function time Fig. 2. Compressive modulus of the different scaffolds RADIATION-CROSSLINKED SCAFFOLDS FROM GELATIN/CM-CHITIN 18 Fig. 3. SEM images and photographs of the scaffolds C. Biodegradation and cytotoxicity of the scaffolds Fig. 4. Enzymatic degradation of the scaffolds in collagenase solution To be considered and applied in tissue engineering as a scaffold, the biodegradability and non-cytotoxicity are fundamental requirements that need to be satisfied. According to Yang et al [11], the collagenase enzyme is commonly used for evaluation of scaffold biodegradability in vitro due to it is able to degrade hydrogels containing proteins. Fig. 4 illustrates the relationship of the biodegradability of the scaffolds with inoculation time. The gained results showed that the scaffolds were to be almost completed degradation by collagenase enzyme activity. After 21 h incubation, the biodegradability of both the scaffolds was around 81 – 83% evenly. However, the biodegradability of the G/CM-chitosan was slightly faster than that of the G/CM-chitin in the first 15 h. The reason could be explained that the swelling rate and the porosity of the G/CM-chitosan scaffold were moderately higher than that of the G/CM-chitin one, leading to a more favorable invasion of the enzyme [7, 11]. Gelatin G/CM-chitosan G/CM-chitin 0 20 40 60 80 100 0 3 6 9 12 15 18 21 24 Incubated time, h D eg ra d a ti o n i n c o ll a g en a se , % G/CM-chitosan G/CM-chitin DANG VAN PHU et al. 19 Fig. 5. The confocal micrographs of hADSCs cultured in extraction media and MTT tested: 1) Fresh culture medium (control), 2) Latex extract (control +), 3) G/CM-chitosan scaffold extract, and 4) G/CM-chitin scaffold extract Fig. 6. Relative growth rate of hADSCs in extraction media Cytotoxicities of G/CM-chitosan and CM-chitin based scaffolds were assessed according to ISO 10993-5:2009 by incubating hADSCs with the extracts of the scaffolds [7, 9]. As a well-known, the MTT assay is a colorimetric test method based on the color intensity of a purple formazan crystal, which depends on the living cell's metabolic activity. The morphology properties and density of cells from the testing extracts after performing MTT assay are presented in Fig. 5. The morphology and cell density for treatments of the G/CM-chitosan and G/CM-chitin were seemingly unchanged from that of the negative control group, while the cells with shrinkage, flaking off, and almost no forming formazan crystals were found in the positive control (latex extract). From these results, it may be suggested that the G/CM-chitosan and G/CM-chitin scaffold exhibited non-cytotoxicity for the hADSCs. Concerning cell ingrowths, the RGR% values of the hADSCs after an incubation period of 24 h with the different extracts are described in Fig. 6. The RGR values were of 96.2% and 97.1% respectively for G/CM-chitosan and G/CM-chitin samples, appropriated 7.3-fold higher than that of the positive control group. These values could be assorted to scoring 1 (non-cytotoxicity) and qualified [4, 9]. The superb non-cytotoxicity or cytocompatibility of these scaffolds was assigned to the biocompatibility of the gelatin, CM-chitosan, and CM-chitin [11], as well as a suitable 3 D microenvironment provided by the scaffold materials for the hADSCs [3]. 0 20 40 60 80 100 120 140 1 2 3 Samples (scaffolds) R G R , % 2. G/CM-chitosan 1. Latex 3. G/CM-chitin 1 2 3 4 RADIATION-CROSSLINKED SCAFFOLDS FROM GELATIN/CM-CHITIN 20 IV. CONCLUSIONS The mixture scaffolds of gelatin/CM- chitosan and gelatin/CM-chitin were successfully fabricated using radiation- crosslinking combined with freeze-drying. The performance in the same behaviors of ameliorating the inherent properties of the gelatin scaffold by CM-chitosan and CM- chitin was evaluated and compared. Both scaffold materials of gelatin/CM-chitosan and gelatin/CM-chitin possessed the swelling degree, porosity and pore size, mechanical strength, biodegradability, and non-cytotoxicity in suitability for human adipose-derived stem cell culture for soft tissue regeneration. The entire results of this work propound a potential of the scaffolds in the application to hADSC culture. Nevertheless, other prime biological- features, such as proliferation and differentiation of hADSCs on the scaffolds should be further explored. ACKNOWLEDGEMENTS This work was financially supported by the Ministry of Science and Technology (MOST), Vietnam under Project No. “DTCB.05/19/TTNCTK” in the period 2019 – 2020. The authors would like to thank VINATOM, VINAGAMMA, and HCMUS for providing the favorable conditions during project implementation. REFERENCES [1]. Z. Dai, J. Ronholm, Y. Tian, B. Sethi and X. Cao, “Sterilization techniques for biodegradable scaffolds in tissue engineering applications”, J. Tissue Eng., 7, 13p, 2016. [2]. Y. Zhou, L. Xu, X. Zhang, Y. Zhao, S. Wei and M. Zhai, “Radiation synthesis of gelatin/CM-chitosan/β-tricalcium phosphate composite scaffold for bone tissue engineering”, Mater. Sci. Eng. C, 32(4), 994, 2012. [3]. T. Agarwal, R. Narayan, S. Maji et al., “Gelatin/Carboxymethyl chitosan based scaffolds for dermal tissue engineering applicationˮ, Int. J. Biol. Macromol., 93(Pt B), 1499, 2016. [4]. W. Xu, Z. Wang, T. Li et al., “Membranes based on carboxymethyl chitin as potential scaffolds for corneal endothelial transplantation”, Polym. J., 49, 789, 2017. [5]. N. Sagar, V.P. Soni and J.R. Bellare, “Influence of carboxymethyl chitin on stability and biocompatibility of 3D nanohydroxyapatite/gelatin/carboxymethyl chitin composite for bone tissue engineering”, J. Biomed. Mater. Res. Part B, 100B(3), 264, 2011. [6]. Y-H. Tsou, J. Khoneisser, P-C. Huang and X. Xu, “Hydrogel as a bioactive material to regulate stem cell fate”, Bioact. Mater., 1(1), 39, 2016. [7]. K-H. Wu, C. Mai, C-W. Lin, K-C. Yang and J. Yu, “The influence of bubble size on chondrogenic differentiation of adipose-derived stem cells in gelatin microbubble scaffolds”, J. Mater. Chem. B, 6(1), 125, 2017. [8]. H-T. Lu, T-W. Lu, C-H. Chen, K-Y. Lu and F- L. Mi, “Development of nanocomposite scaffolds based on biomineralization of N,O- carboxymethyl chitosan/fucoidan conjugates for bone tissue engineering”, Int. J. Biol. Macromol., 120(Pt B), 2335, 2018. [9]. N.T.T. Thao, T.M. Quan, H.D. Thao, T.C. Toai and T.L.B. Ha, “Gelatin-alginate sponge: A potential scaffold for adipose tissue engineering”, Eur. J. Biomed. Pharm. Sci., 2(7), 48, 2015. DANG VAN PHU et al. 21 [10]. D.V. Phu, B.D. Du, L.N.A. Tuan, T.L.B. Ha and N.Q. Hien, “Synthesis of radiation crosslinked gelatin/carboxymethyl chitosan hydrogel scaffold for tissue culture”, Vietnam J. Chem., 57(4e1,2), 223, 2019. [11]. C. Yang, L. Xu, Y. Zhou et al., “A green fabrication approach of gelatin/CM-chitosan hybrid hydrogel for wound healing”, Carbohydr. Polym., 82(4), 1297, 2010. [12]. X. Huang, Y. Zhang, X. Zhang, L. Xu, X. Chen and S. Wei, “Influence of radiation crosslinked carboxymethyl-chitosan/gelatin hydrogel on cutaneous wound healing”, Mater. Sci. Eng. C, 33(8), 4816, 2013. [13]. L. Zhao, H. Mitomo, N. Nagasawa, F. Yoshii and T. Kume, “Radiation synthesis and characteristic of the hydrogels based on carboxymethylated chitin derivatives”, Carbohydr. Polym., 51(2), 169, 2003.
File đính kèm:
radiation_crosslinked_scaffolds_from_gelatincm_chitin_and_ge.pdf