Performance comparison of dynamic elastic optical networks with optical regeneration
Abstract: We have investigated optical regeneration issues and application in elastic optical networks that are capable of providing dynamically optical paths with flexible bandwidths. We have analyzed the impact of optical regeneration in elastic optical networks and clarified various usage scenarios. We have then evaluated and compared the performance, in terms of the overall blocking probability and the total accommodated traffic volume, of three possible network scenarios with regeneration capability including (i) no regeneration, (ii) 3R regeneration, and (iii) 4R regeneration for practical network topologies. Numerical simulation proved that deployment of optical regeneration devices can exploit elastic optical networking to enhance the network performance for provisioning dynamically bandwidth-Flexible lightpath services. It is also demonstrated that using re-modulation function while regenerating optical signals (4R regeneration) can further improve the network performance. However, due to the high cost of optical regeneration devices, especially all-optical ones, and more functional regenerators, the trade-off between the performance enhancement and the necessary number of regenerating devices needs to be carefully considered
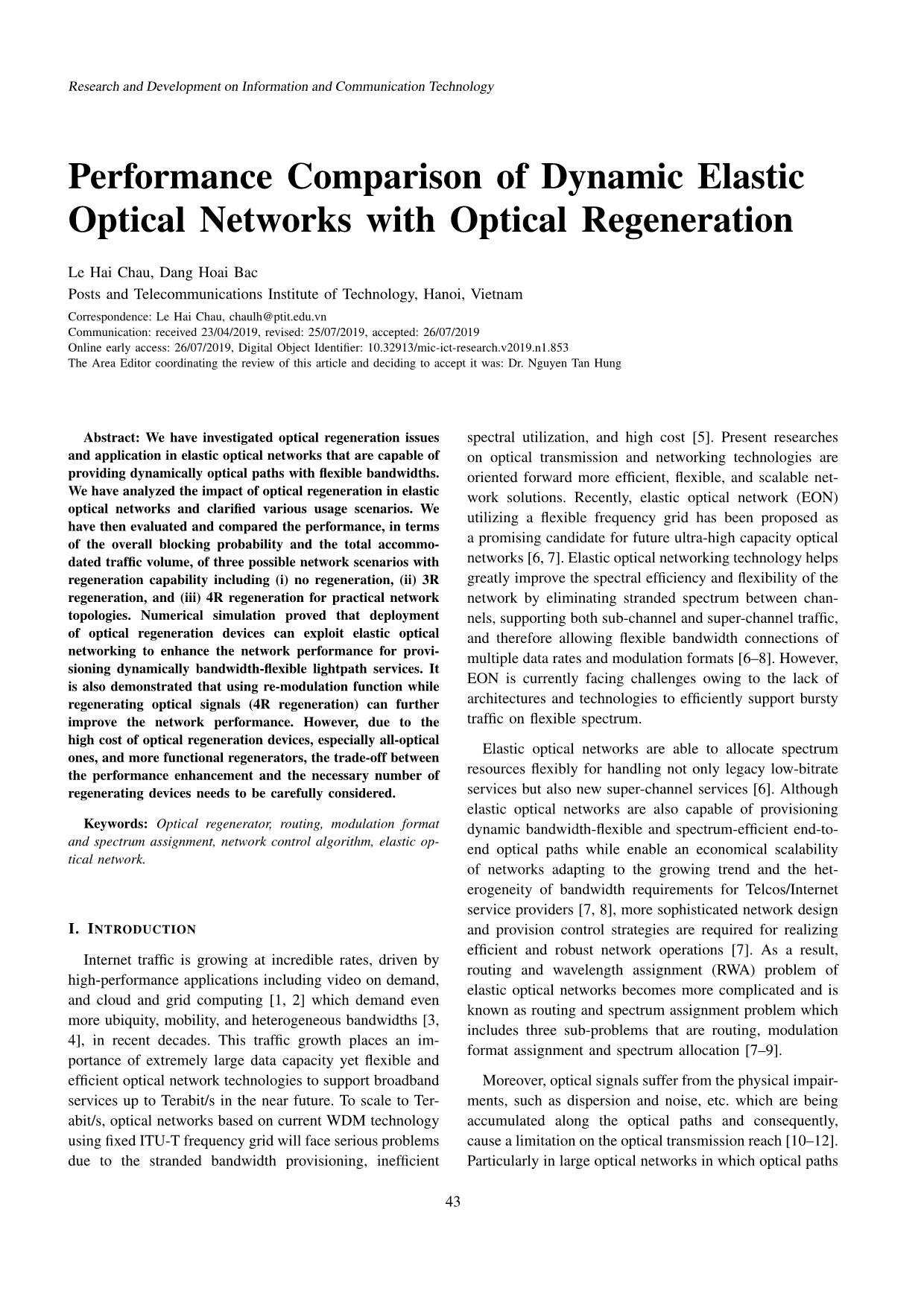
Trang 1
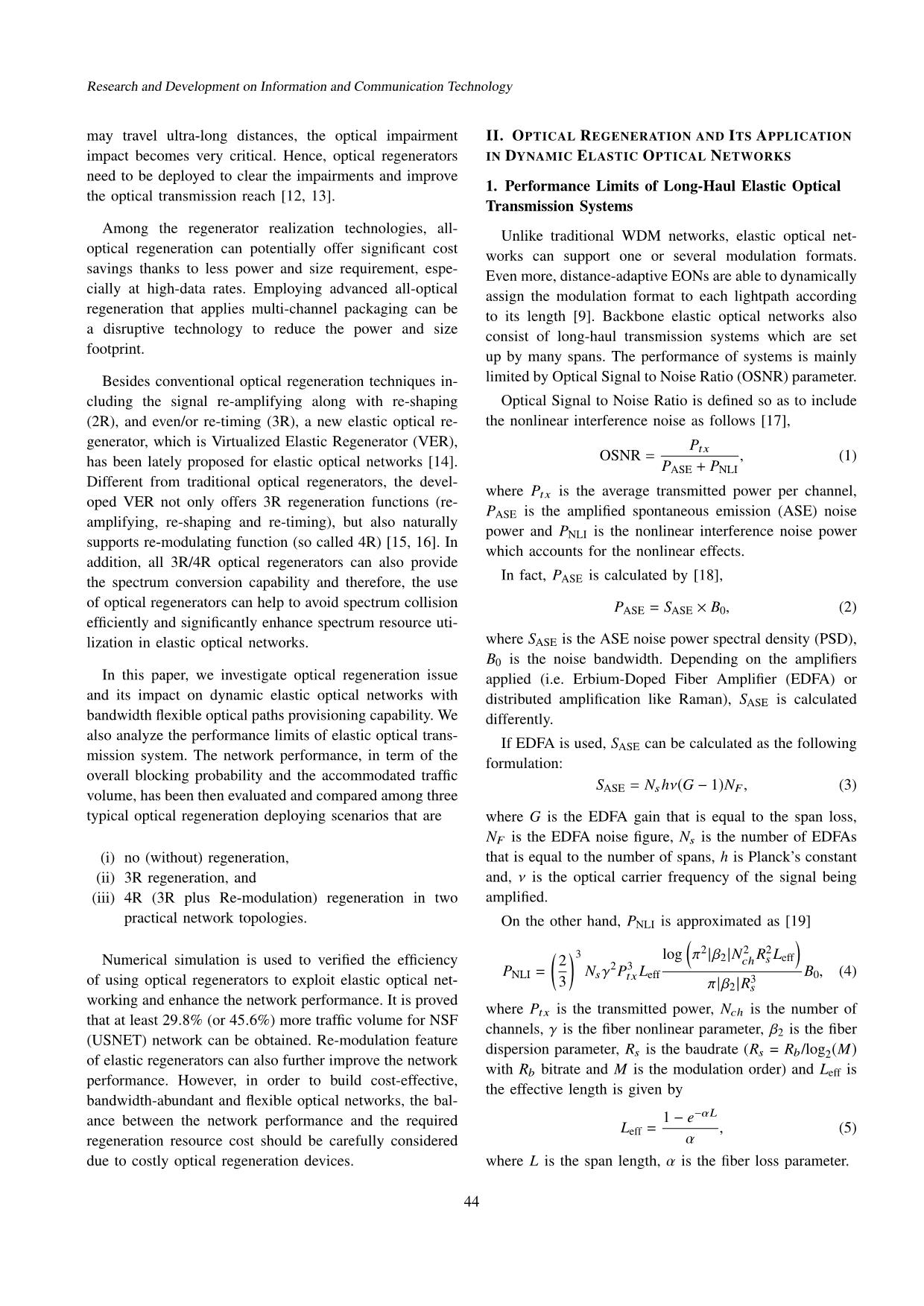
Trang 2
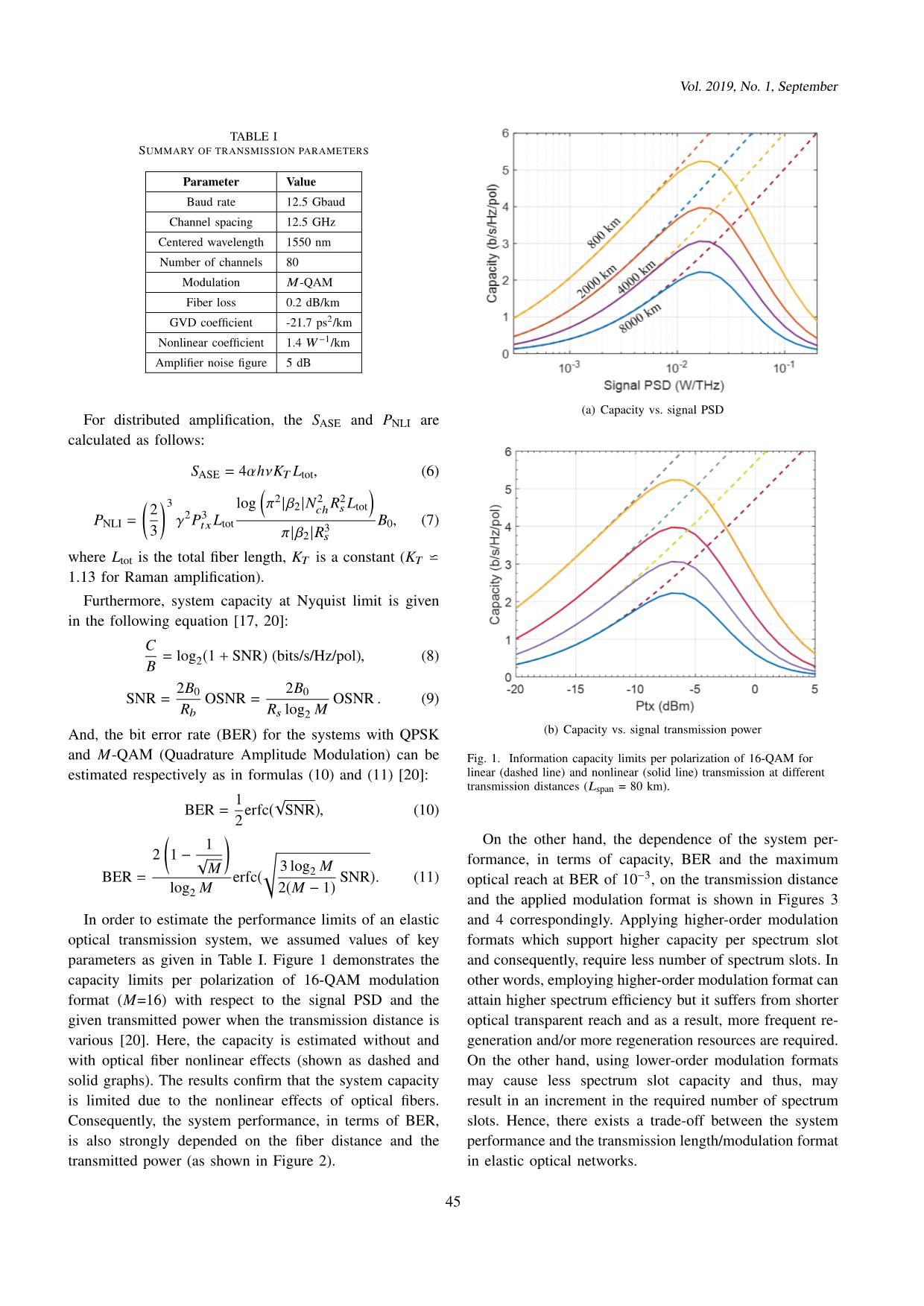
Trang 3
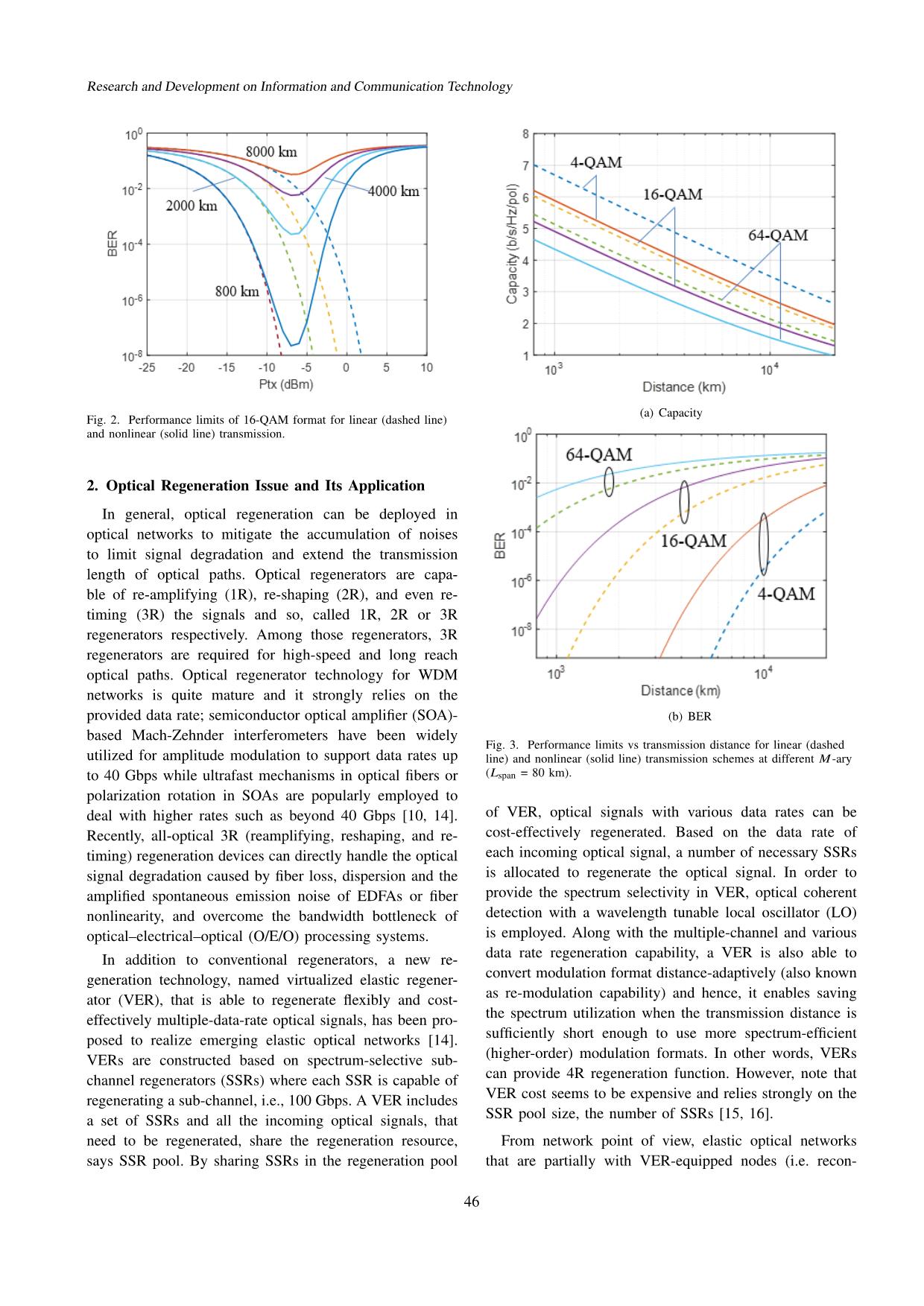
Trang 4
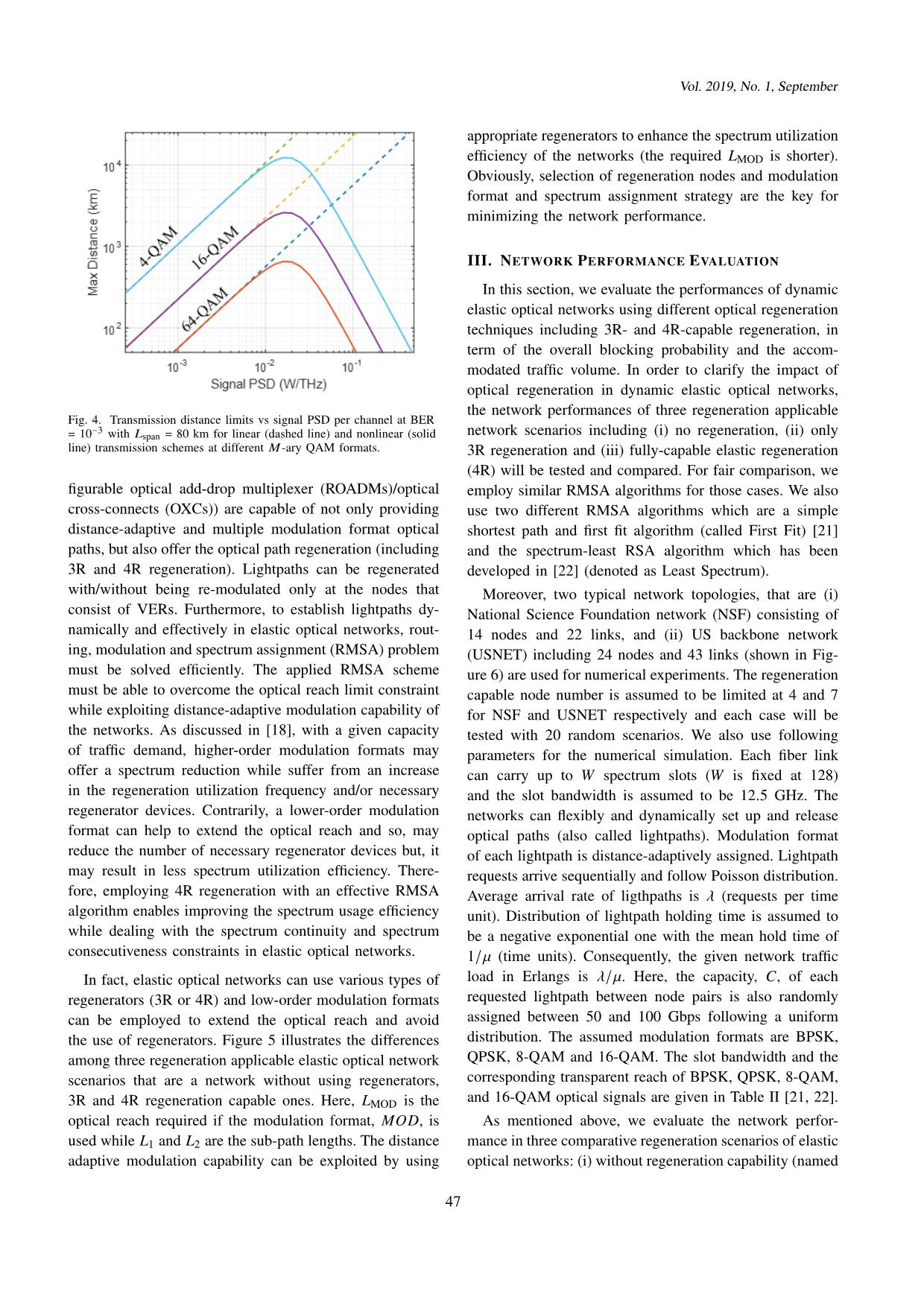
Trang 5
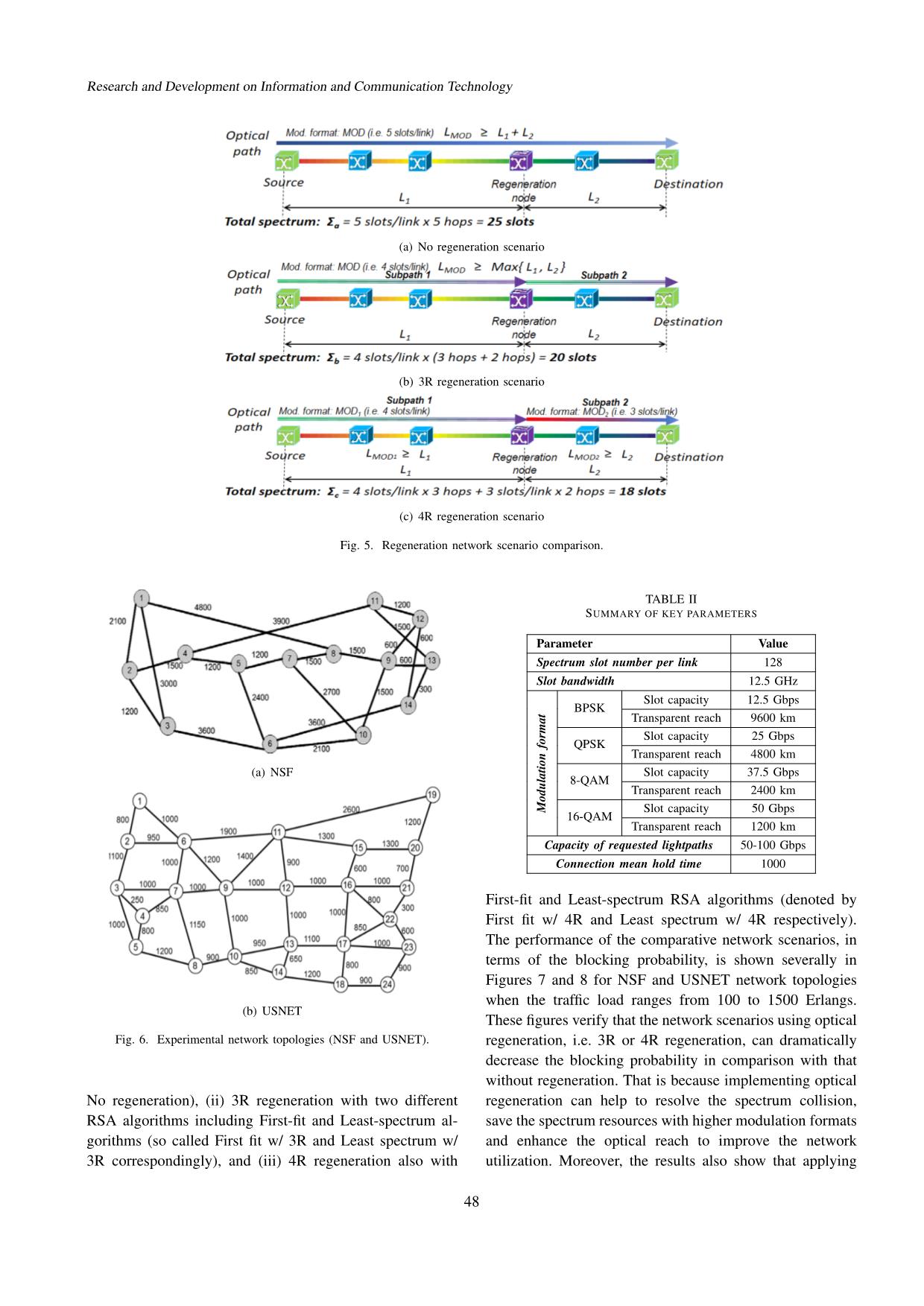
Trang 6
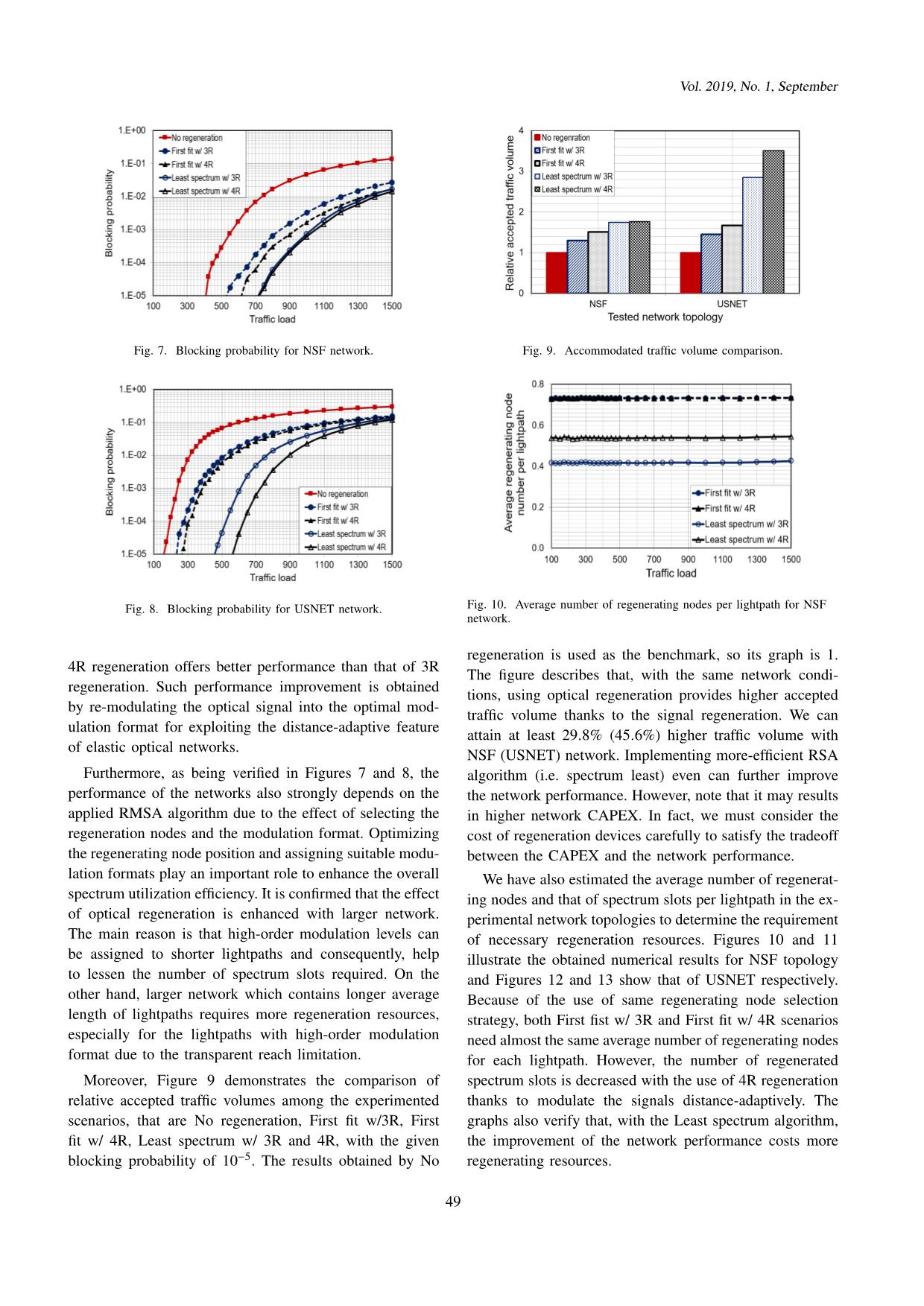
Trang 7
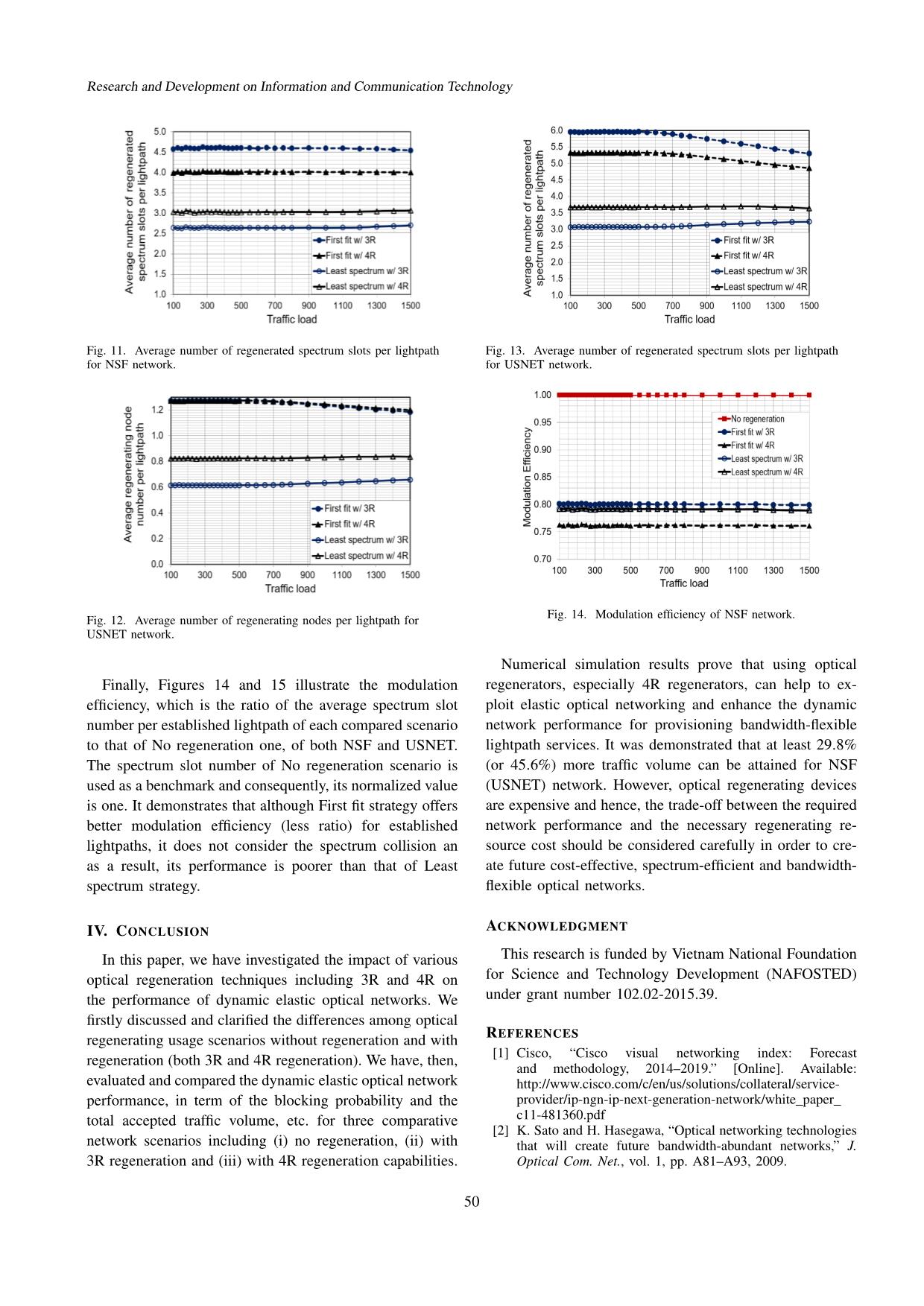
Trang 8
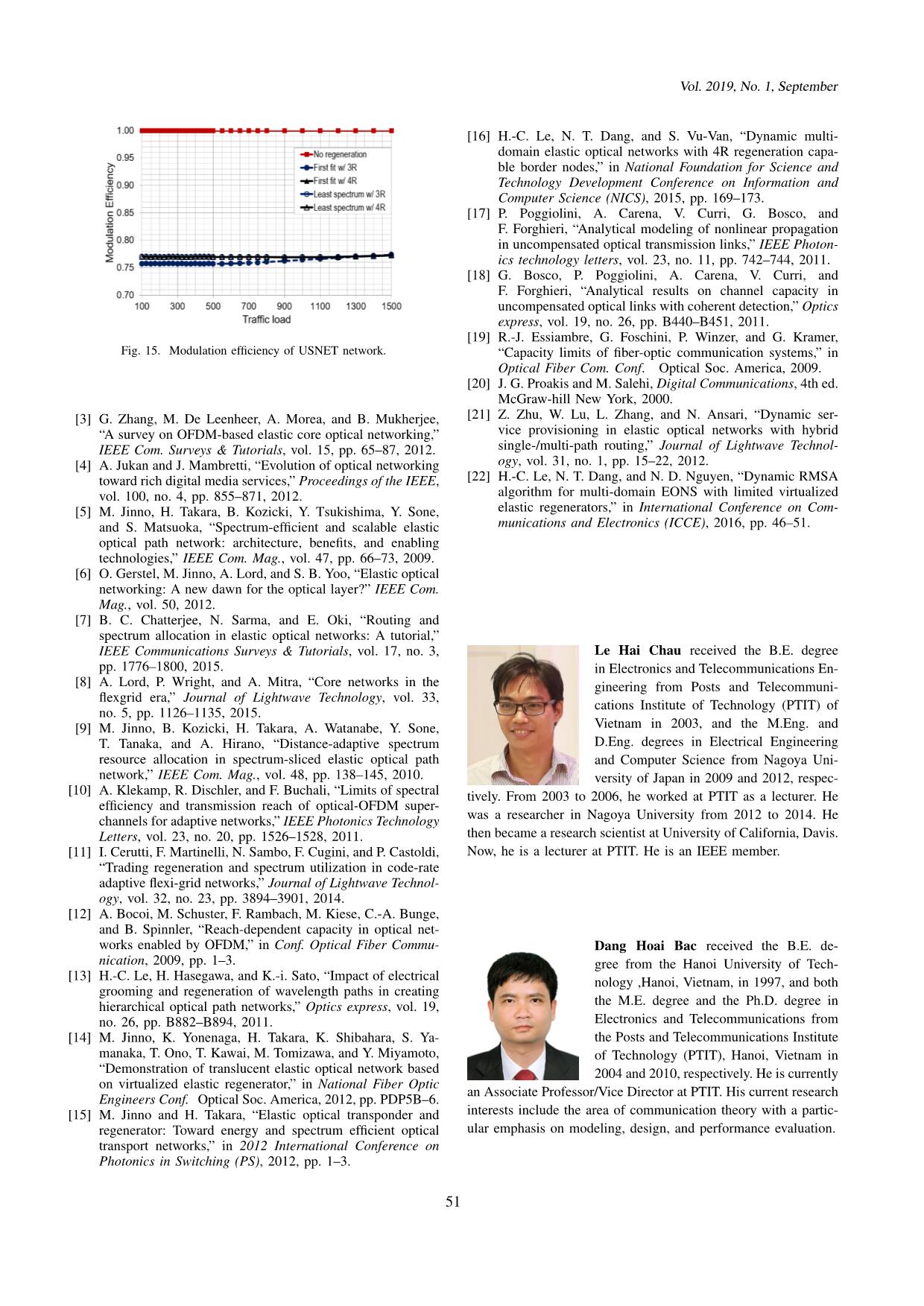
Trang 9
Tóm tắt nội dung tài liệu: Performance comparison of dynamic elastic optical networks with optical regeneration
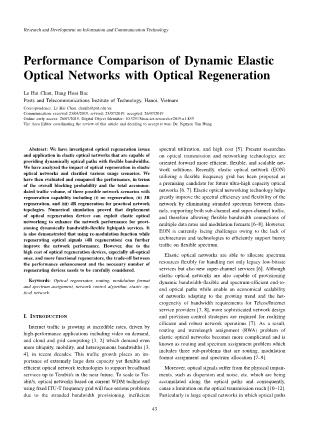
h and first fit algorithm (called First Fit) [21] and the spectrum-least RSA algorithm which has been developed in [22] (denoted as Least Spectrum). Moreover, two typical network topologies, that are (i) National Science Foundation network (NSF) consisting of 14 nodes and 22 links, and (ii) US backbone network (USNET) including 24 nodes and 43 links (shown in Fig- ure 6) are used for numerical experiments. The regeneration capable node number is assumed to be limited at 4 and 7 for NSF and USNET respectively and each case will be tested with 20 random scenarios. We also use following parameters for the numerical simulation. Each fiber link can carry up to W spectrum slots (W is fixed at 128) and the slot bandwidth is assumed to be 12.5 GHz. The networks can flexibly and dynamically set up and release optical paths (also called lightpaths). Modulation format of each lightpath is distance-adaptively assigned. Lightpath requests arrive sequentially and follow Poisson distribution. Average arrival rate of ligthpaths is λ (requests per time unit). Distribution of lightpath holding time is assumed to be a negative exponential one with the mean hold time of 1/µ (time units). Consequently, the given network traffic load in Erlangs is λ/µ. Here, the capacity, C, of each requested lightpath between node pairs is also randomly assigned between 50 and 100 Gbps following a uniform distribution. The assumed modulation formats are BPSK, QPSK, 8-QAM and 16-QAM. The slot bandwidth and the corresponding transparent reach of BPSK, QPSK, 8-QAM, and 16-QAM optical signals are given in Table II [21, 22]. As mentioned above, we evaluate the network perfor- mance in three comparative regeneration scenarios of elastic optical networks: (i) without regeneration capability (named 47 Research and Development on Information and Communication Technology (a) No regeneration scenario (b) 3R regeneration scenario (c) 4R regeneration scenario Fig. 5. Regeneration network scenario comparison. (a) NSF (b) USNET Fig. 6. Experimental network topologies (NSF and USNET). No regeneration), (ii) 3R regeneration with two different RSA algorithms including First-fit and Least-spectrum al- gorithms (so called First fit w/ 3R and Least spectrum w/ 3R correspondingly), and (iii) 4R regeneration also with TABLE II SUMMARY OF KEY PARAMETERS Parameter Value Spectrum slot number per link 128 Slot bandwidth 12.5 GHz M od ul at io n fo rm at BPSK Slot capacity 12.5 Gbps Transparent reach 9600 km QPSK Slot capacity 25 Gbps Transparent reach 4800 km 8-QAM Slot capacity 37.5 Gbps Transparent reach 2400 km 16-QAM Slot capacity 50 Gbps Transparent reach 1200 km Capacity of requested lightpaths 50-100 Gbps Connection mean hold time 1000 First-fit and Least-spectrum RSA algorithms (denoted by First fit w/ 4R and Least spectrum w/ 4R respectively). The performance of the comparative network scenarios, in terms of the blocking probability, is shown severally in Figures 7 and 8 for NSF and USNET network topologies when the traffic load ranges from 100 to 1500 Erlangs. These figures verify that the network scenarios using optical regeneration, i.e. 3R or 4R regeneration, can dramatically decrease the blocking probability in comparison with that without regeneration. That is because implementing optical regeneration can help to resolve the spectrum collision, save the spectrum resources with higher modulation formats and enhance the optical reach to improve the network utilization. Moreover, the results also show that applying 48 Vol. 2019, No. 1, September Fig. 7. Blocking probability for NSF network. Fig. 8. Blocking probability for USNET network. 4R regeneration offers better performance than that of 3R regeneration. Such performance improvement is obtained by re-modulating the optical signal into the optimal mod- ulation format for exploiting the distance-adaptive feature of elastic optical networks. Furthermore, as being verified in Figures 7 and 8, the performance of the networks also strongly depends on the applied RMSA algorithm due to the effect of selecting the regeneration nodes and the modulation format. Optimizing the regenerating node position and assigning suitable modu- lation formats play an important role to enhance the overall spectrum utilization efficiency. It is confirmed that the effect of optical regeneration is enhanced with larger network. The main reason is that high-order modulation levels can be assigned to shorter lightpaths and consequently, help to lessen the number of spectrum slots required. On the other hand, larger network which contains longer average length of lightpaths requires more regeneration resources, especially for the lightpaths with high-order modulation format due to the transparent reach limitation. Moreover, Figure 9 demonstrates the comparison of relative accepted traffic volumes among the experimented scenarios, that are No regeneration, First fit w/3R, First fit w/ 4R, Least spectrum w/ 3R and 4R, with the given blocking probability of 10−5. The results obtained by No Fig. 9. Accommodated traffic volume comparison. Fig. 10. Average number of regenerating nodes per lightpath for NSF network. regeneration is used as the benchmark, so its graph is 1. The figure describes that, with the same network condi- tions, using optical regeneration provides higher accepted traffic volume thanks to the signal regeneration. We can attain at least 29.8% (45.6%) higher traffic volume with NSF (USNET) network. Implementing more-efficient RSA algorithm (i.e. spectrum least) even can further improve the network performance. However, note that it may results in higher network CAPEX. In fact, we must consider the cost of regeneration devices carefully to satisfy the tradeoff between the CAPEX and the network performance. We have also estimated the average number of regenerat- ing nodes and that of spectrum slots per lightpath in the ex- perimental network topologies to determine the requirement of necessary regeneration resources. Figures 10 and 11 illustrate the obtained numerical results for NSF topology and Figures 12 and 13 show that of USNET respectively. Because of the use of same regenerating node selection strategy, both First fist w/ 3R and First fit w/ 4R scenarios need almost the same average number of regenerating nodes for each lightpath. However, the number of regenerated spectrum slots is decreased with the use of 4R regeneration thanks to modulate the signals distance-adaptively. The graphs also verify that, with the Least spectrum algorithm, the improvement of the network performance costs more regenerating resources. 49 Research and Development on Information and Communication Technology Fig. 11. Average number of regenerated spectrum slots per lightpath for NSF network. Fig. 12. Average number of regenerating nodes per lightpath for USNET network. Finally, Figures 14 and 15 illustrate the modulation efficiency, which is the ratio of the average spectrum slot number per established lightpath of each compared scenario to that of No regeneration one, of both NSF and USNET. The spectrum slot number of No regeneration scenario is used as a benchmark and consequently, its normalized value is one. It demonstrates that although First fit strategy offers better modulation efficiency (less ratio) for established lightpaths, it does not consider the spectrum collision an as a result, its performance is poorer than that of Least spectrum strategy. IV. CONCLUSION In this paper, we have investigated the impact of various optical regeneration techniques including 3R and 4R on the performance of dynamic elastic optical networks. We firstly discussed and clarified the differences among optical regenerating usage scenarios without regeneration and with regeneration (both 3R and 4R regeneration). We have, then, evaluated and compared the dynamic elastic optical network performance, in term of the blocking probability and the total accepted traffic volume, etc. for three comparative network scenarios including (i) no regeneration, (ii) with 3R regeneration and (iii) with 4R regeneration capabilities. Fig. 13. Average number of regenerated spectrum slots per lightpath for USNET network. Fig. 14. Modulation efficiency of NSF network. Numerical simulation results prove that using optical regenerators, especially 4R regenerators, can help to ex- ploit elastic optical networking and enhance the dynamic network performance for provisioning bandwidth-flexible lightpath services. It was demonstrated that at least 29.8% (or 45.6%) more traffic volume can be attained for NSF (USNET) network. However, optical regenerating devices are expensive and hence, the trade-off between the required network performance and the necessary regenerating re- source cost should be considered carefully in order to cre- ate future cost-effective, spectrum-efficient and bandwidth- flexible optical networks. ACKNOWLEDGMENT This research is funded by Vietnam National Foundation for Science and Technology Development (NAFOSTED) under grant number 102.02-2015.39. REFERENCES [1] Cisco, “Cisco visual networking index: Forecast and methodology, 2014–2019.” [Online]. Available: provider/ip-ngn-ip-next-generation-network/white paper c11-481360.pdf [2] K. Sato and H. Hasegawa, “Optical networking technologies that will create future bandwidth-abundant networks,” J. Optical Com. Net., vol. 1, pp. A81–A93, 2009. 50 Vol. 2019, No. 1, September Fig. 15. Modulation efficiency of USNET network. [3] G. Zhang, M. De Leenheer, A. Morea, and B. Mukherjee, “A survey on OFDM-based elastic core optical networking,” IEEE Com. Surveys & Tutorials, vol. 15, pp. 65–87, 2012. [4] A. Jukan and J. Mambretti, “Evolution of optical networking toward rich digital media services,” Proceedings of the IEEE, vol. 100, no. 4, pp. 855–871, 2012. [5] M. Jinno, H. Takara, B. Kozicki, Y. Tsukishima, Y. Sone, and S. Matsuoka, “Spectrum-efficient and scalable elastic optical path network: architecture, benefits, and enabling technologies,” IEEE Com. Mag., vol. 47, pp. 66–73, 2009. [6] O. Gerstel, M. Jinno, A. Lord, and S. B. Yoo, “Elastic optical networking: A new dawn for the optical layer?” IEEE Com. Mag., vol. 50, 2012. [7] B. C. Chatterjee, N. Sarma, and E. Oki, “Routing and spectrum allocation in elastic optical networks: A tutorial,” IEEE Communications Surveys & Tutorials, vol. 17, no. 3, pp. 1776–1800, 2015. [8] A. Lord, P. Wright, and A. Mitra, “Core networks in the flexgrid era,” Journal of Lightwave Technology, vol. 33, no. 5, pp. 1126–1135, 2015. [9] M. Jinno, B. Kozicki, H. Takara, A. Watanabe, Y. Sone, T. Tanaka, and A. Hirano, “Distance-adaptive spectrum resource allocation in spectrum-sliced elastic optical path network,” IEEE Com. Mag., vol. 48, pp. 138–145, 2010. [10] A. Klekamp, R. Dischler, and F. Buchali, “Limits of spectral efficiency and transmission reach of optical-OFDM super- channels for adaptive networks,” IEEE Photonics Technology Letters, vol. 23, no. 20, pp. 1526–1528, 2011. [11] I. Cerutti, F. Martinelli, N. Sambo, F. Cugini, and P. Castoldi, “Trading regeneration and spectrum utilization in code-rate adaptive flexi-grid networks,” Journal of Lightwave Technol- ogy, vol. 32, no. 23, pp. 3894–3901, 2014. [12] A. Bocoi, M. Schuster, F. Rambach, M. Kiese, C.-A. Bunge, and B. Spinnler, “Reach-dependent capacity in optical net- works enabled by OFDM,” in Conf. Optical Fiber Commu- nication, 2009, pp. 1–3. [13] H.-C. Le, H. Hasegawa, and K.-i. Sato, “Impact of electrical grooming and regeneration of wavelength paths in creating hierarchical optical path networks,” Optics express, vol. 19, no. 26, pp. B882–B894, 2011. [14] M. Jinno, K. Yonenaga, H. Takara, K. Shibahara, S. Ya- manaka, T. Ono, T. Kawai, M. Tomizawa, and Y. Miyamoto, “Demonstration of translucent elastic optical network based on virtualized elastic regenerator,” in National Fiber Optic Engineers Conf. Optical Soc. America, 2012, pp. PDP5B–6. [15] M. Jinno and H. Takara, “Elastic optical transponder and regenerator: Toward energy and spectrum efficient optical transport networks,” in 2012 International Conference on Photonics in Switching (PS), 2012, pp. 1–3. [16] H.-C. Le, N. T. Dang, and S. Vu-Van, “Dynamic multi- domain elastic optical networks with 4R regeneration capa- ble border nodes,” in National Foundation for Science and Technology Development Conference on Information and Computer Science (NICS), 2015, pp. 169–173. [17] P. Poggiolini, A. Carena, V. Curri, G. Bosco, and F. Forghieri, “Analytical modeling of nonlinear propagation in uncompensated optical transmission links,” IEEE Photon- ics technology letters, vol. 23, no. 11, pp. 742–744, 2011. [18] G. Bosco, P. Poggiolini, A. Carena, V. Curri, and F. Forghieri, “Analytical results on channel capacity in uncompensated optical links with coherent detection,” Optics express, vol. 19, no. 26, pp. B440–B451, 2011. [19] R.-J. Essiambre, G. Foschini, P. Winzer, and G. Kramer, “Capacity limits of fiber-optic communication systems,” in Optical Fiber Com. Conf. Optical Soc. America, 2009. [20] J. G. Proakis and M. Salehi, Digital Communications, 4th ed. McGraw-hill New York, 2000. [21] Z. Zhu, W. Lu, L. Zhang, and N. Ansari, “Dynamic ser- vice provisioning in elastic optical networks with hybrid single-/multi-path routing,” Journal of Lightwave Technol- ogy, vol. 31, no. 1, pp. 15–22, 2012. [22] H.-C. Le, N. T. Dang, and N. D. Nguyen, “Dynamic RMSA algorithm for multi-domain EONS with limited virtualized elastic regenerators,” in International Conference on Com- munications and Electronics (ICCE), 2016, pp. 46–51. Le Hai Chau received the B.E. degree in Electronics and Telecommunications En- gineering from Posts and Telecommuni- cations Institute of Technology (PTIT) of Vietnam in 2003, and the M.Eng. and D.Eng. degrees in Electrical Engineering and Computer Science from Nagoya Uni- versity of Japan in 2009 and 2012, respec- tively. From 2003 to 2006, he worked at PTIT as a lecturer. He was a researcher in Nagoya University from 2012 to 2014. He then became a research scientist at University of California, Davis. Now, he is a lecturer at PTIT. He is an IEEE member. Dang Hoai Bac received the B.E. de- gree from the Hanoi University of Tech- nology ,Hanoi, Vietnam, in 1997, and both the M.E. degree and the Ph.D. degree in Electronics and Telecommunications from the Posts and Telecommunications Institute of Technology (PTIT), Hanoi, Vietnam in 2004 and 2010, respectively. He is currently an Associate Professor/Vice Director at PTIT. His current research interests include the area of communication theory with a partic- ular emphasis on modeling, design, and performance evaluation. 51
File đính kèm:
performance_comparison_of_dynamic_elastic_optical_networks_w.pdf