Highly photocatalytic activity of natural halloysite - Based material for the treatment of dyes in wastewater
In this study, the halloysite nanotube material will be fabricated from a
natural halloysite mineral and used as a support for the photocatalytic
activity phase based on TiO2. The material is characterized by modern
physicochemical methods such as XRD, SEM, BET, UV - vis spectrum, and
EDX. Accordingly, the refined halloysite has a nanoscale with a length of
about 1.3 μm and a capillary size of about 5 nm. After the deposition of Ag
- TiO2 on the halloysite, the specific surface of the material measured by
the BET method was about 60 m2/g, and the structure of the halloysite
was intact. The band - gap energy of as - prepared materials is also
significantly improved in comparison to pure TiO2, makes the material
capable of absorbing longer wavelengths of light in the photocatalytic
process. The Photocatalyst based on Halloysite and TiO2 showed very high
efficiency, up to more than 95% in the decomposition of typical organic
pollutant RR - 195. This result shows great potential in this novel material
in environmental treatment applications.
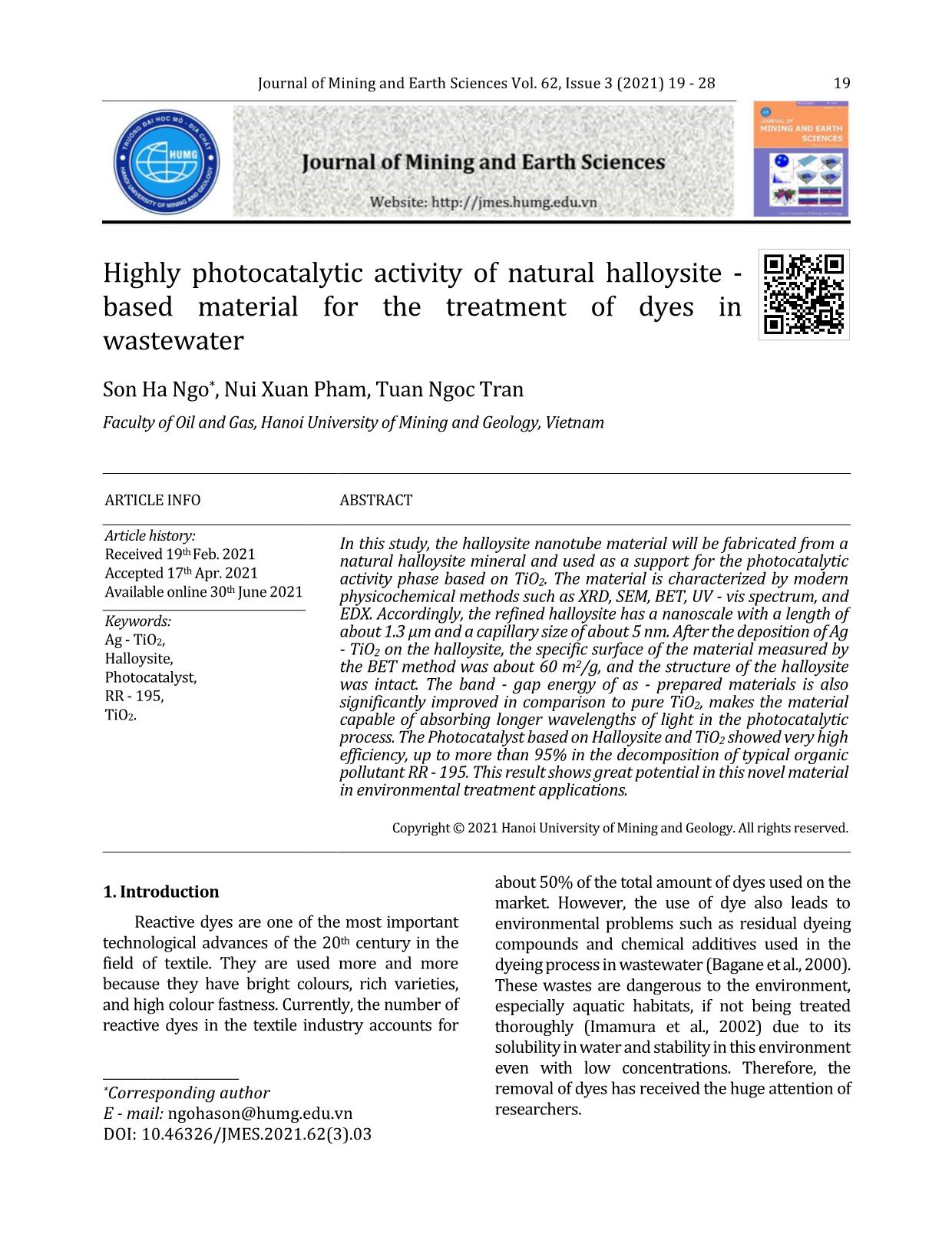
Trang 1
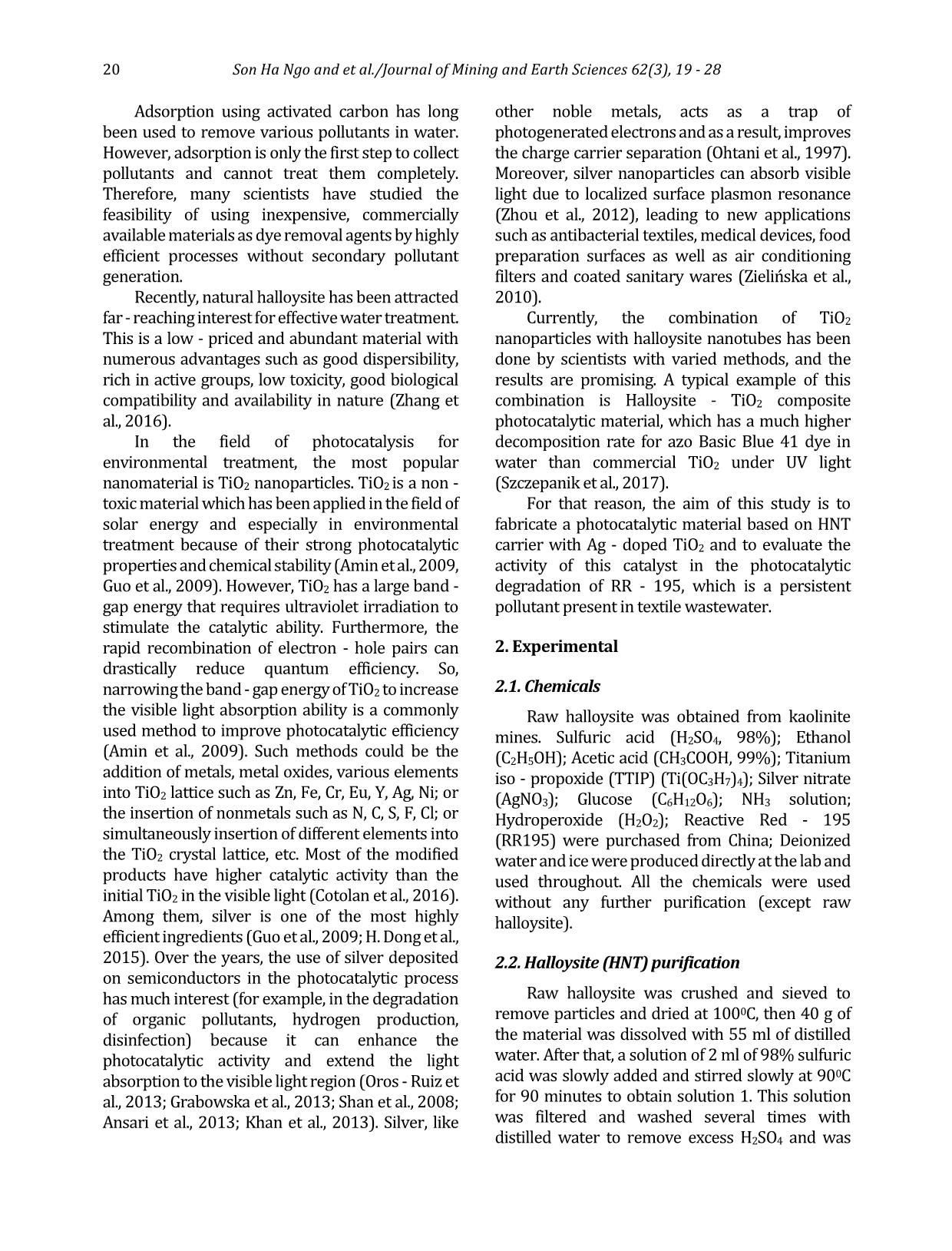
Trang 2
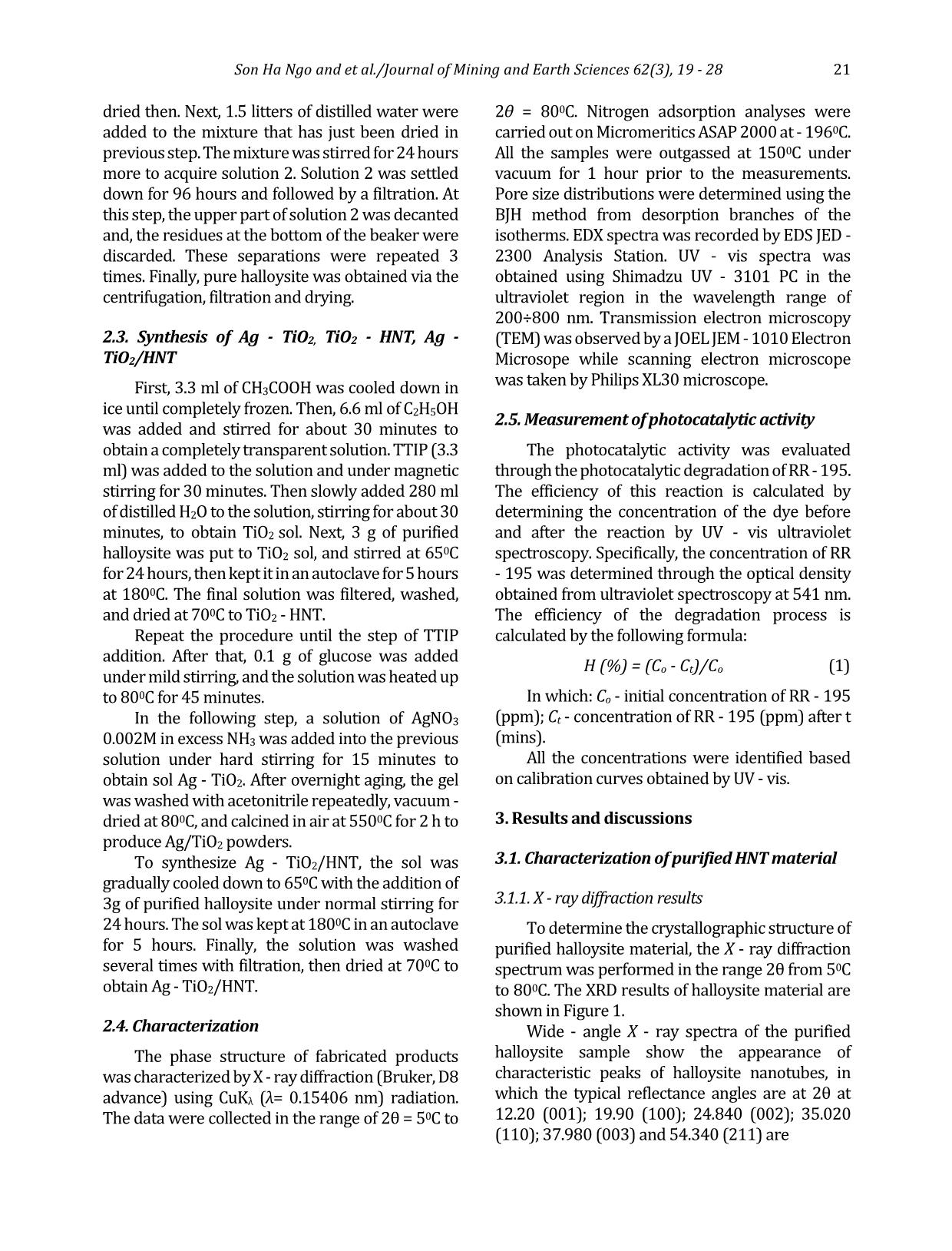
Trang 3
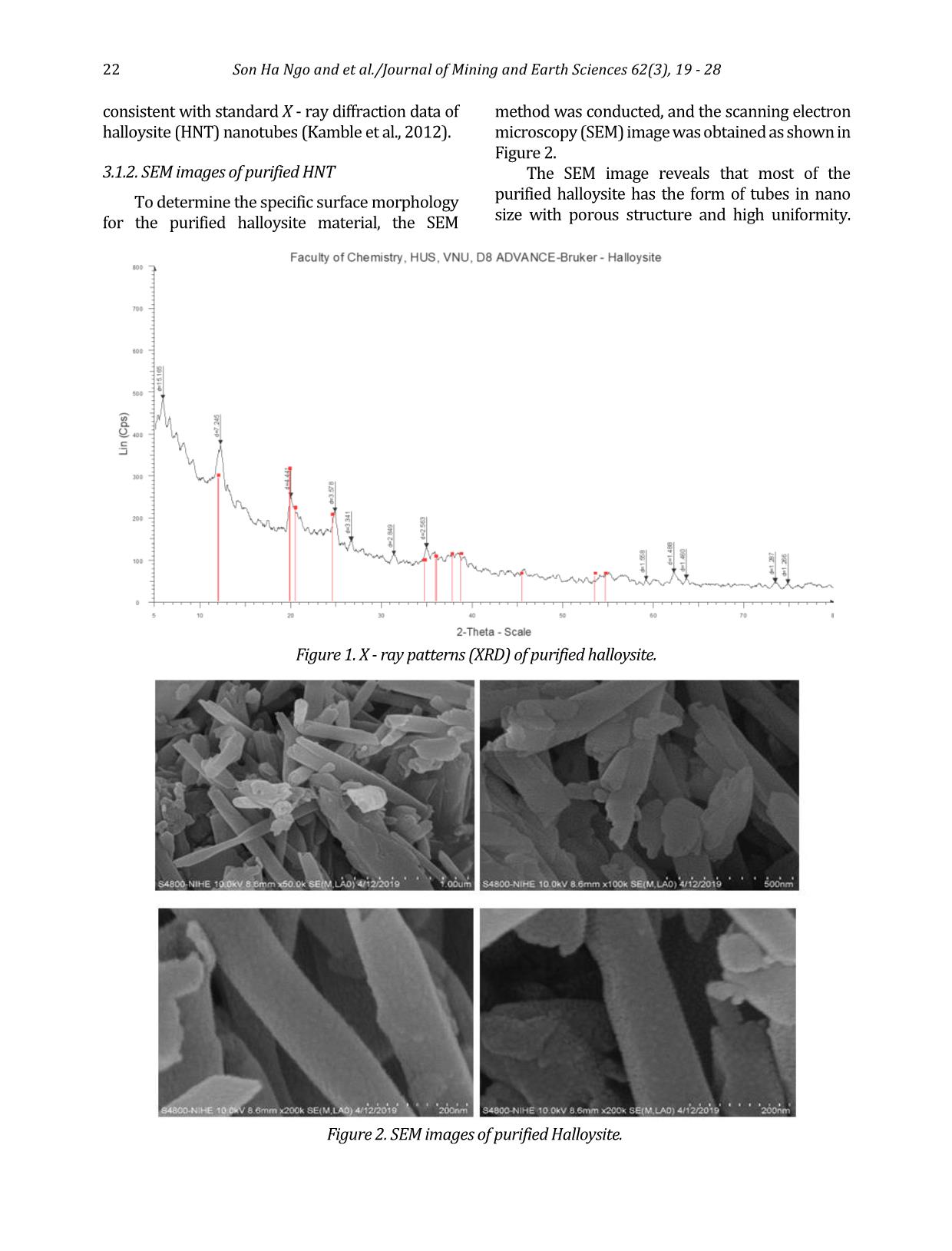
Trang 4
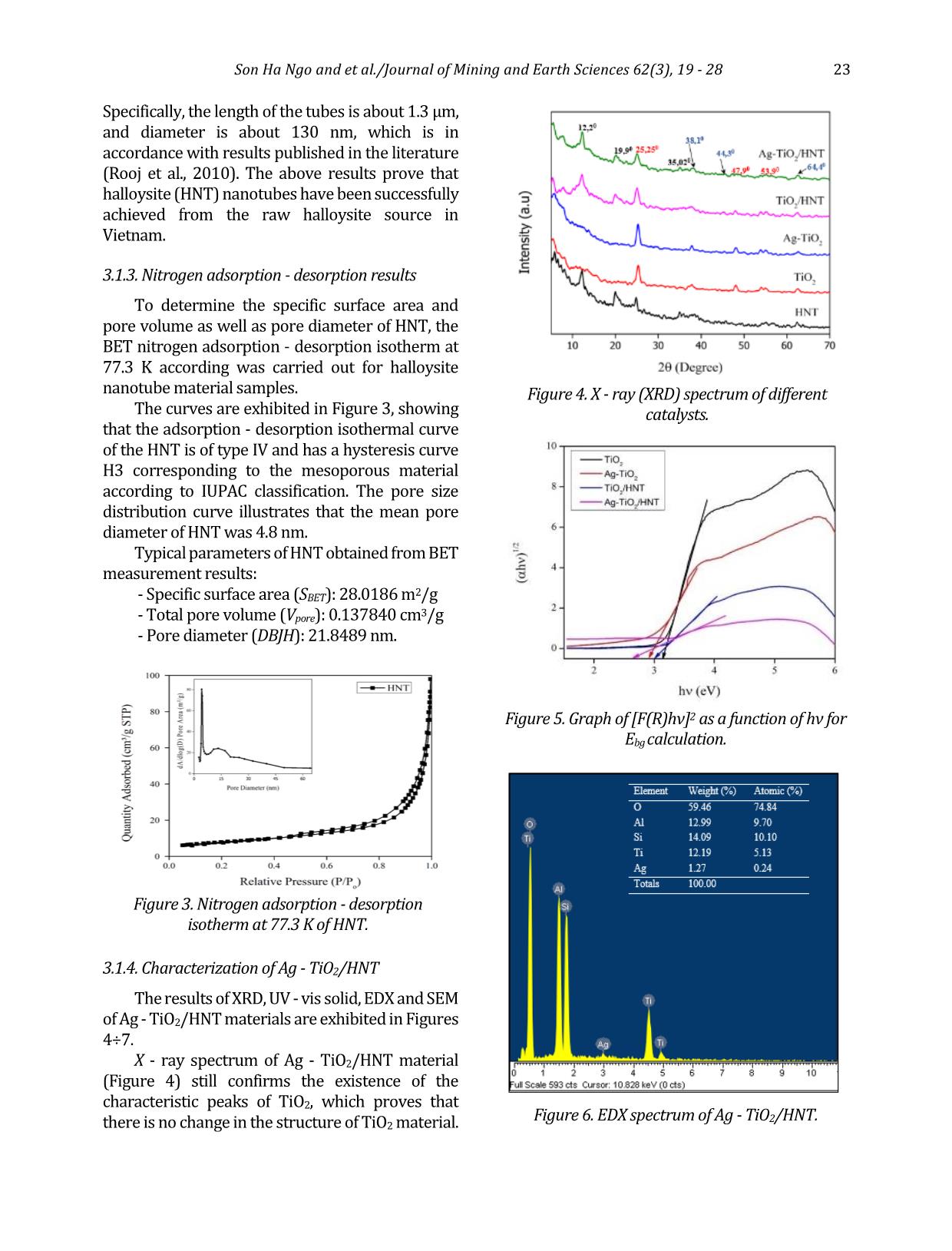
Trang 5
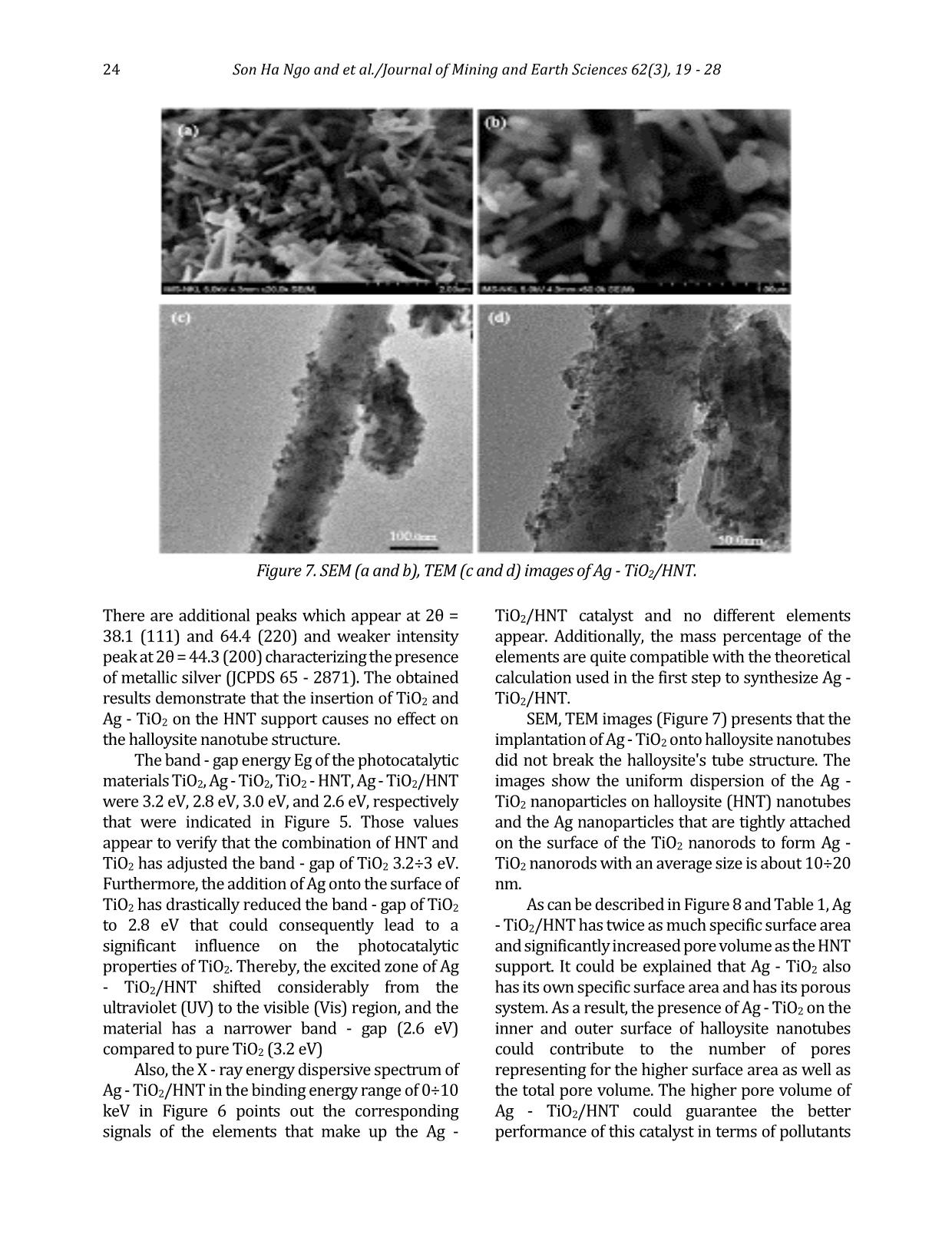
Trang 6
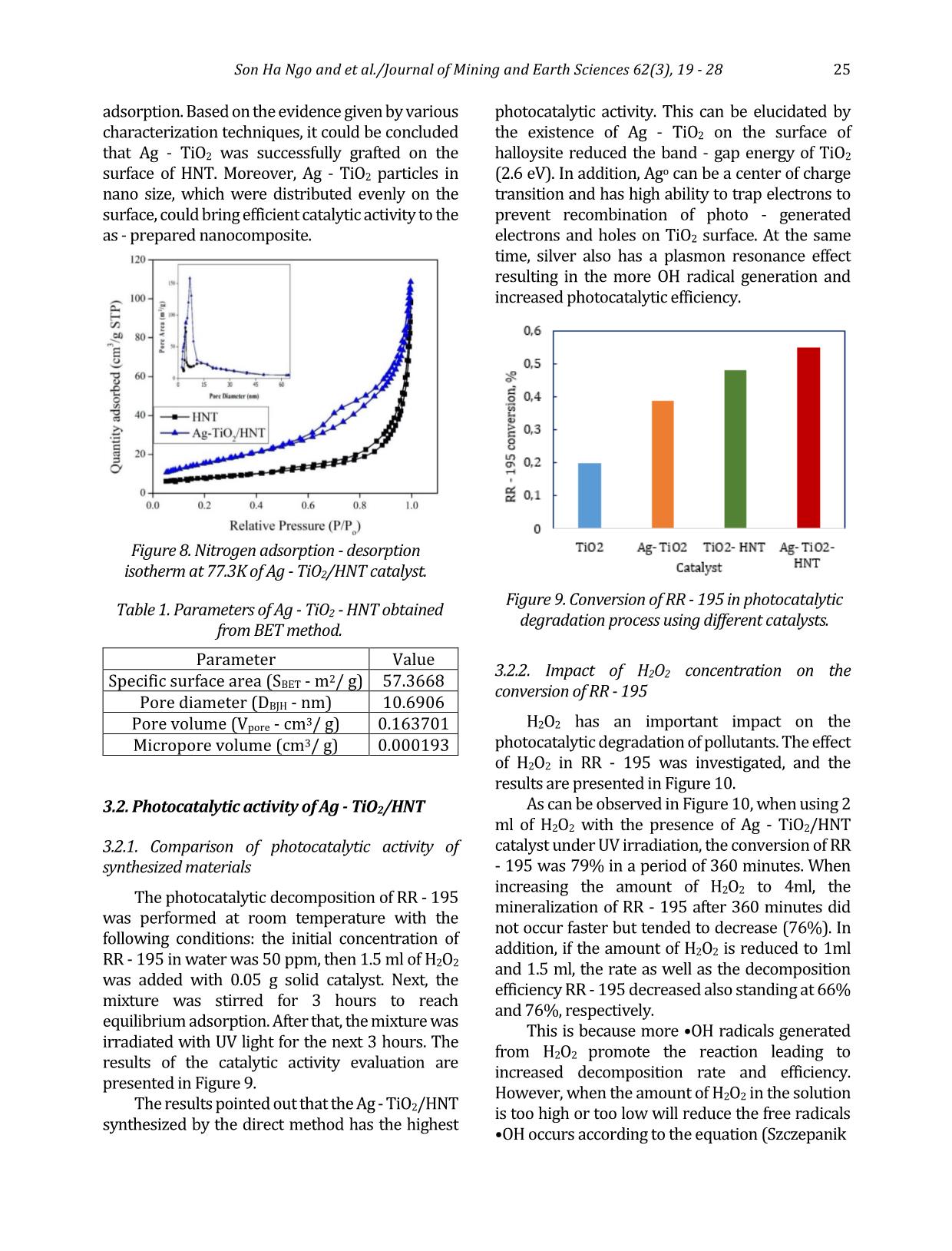
Trang 7
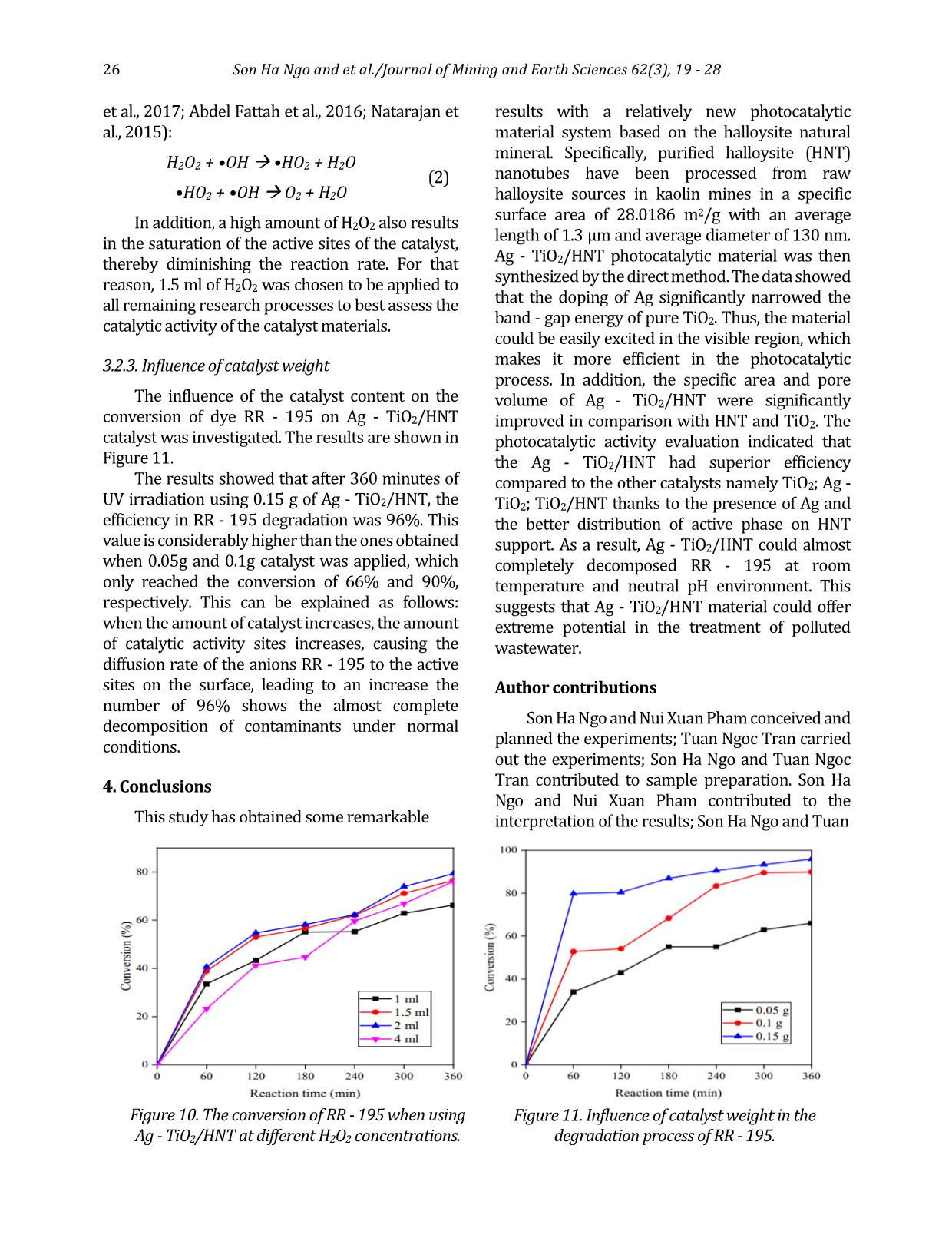
Trang 8
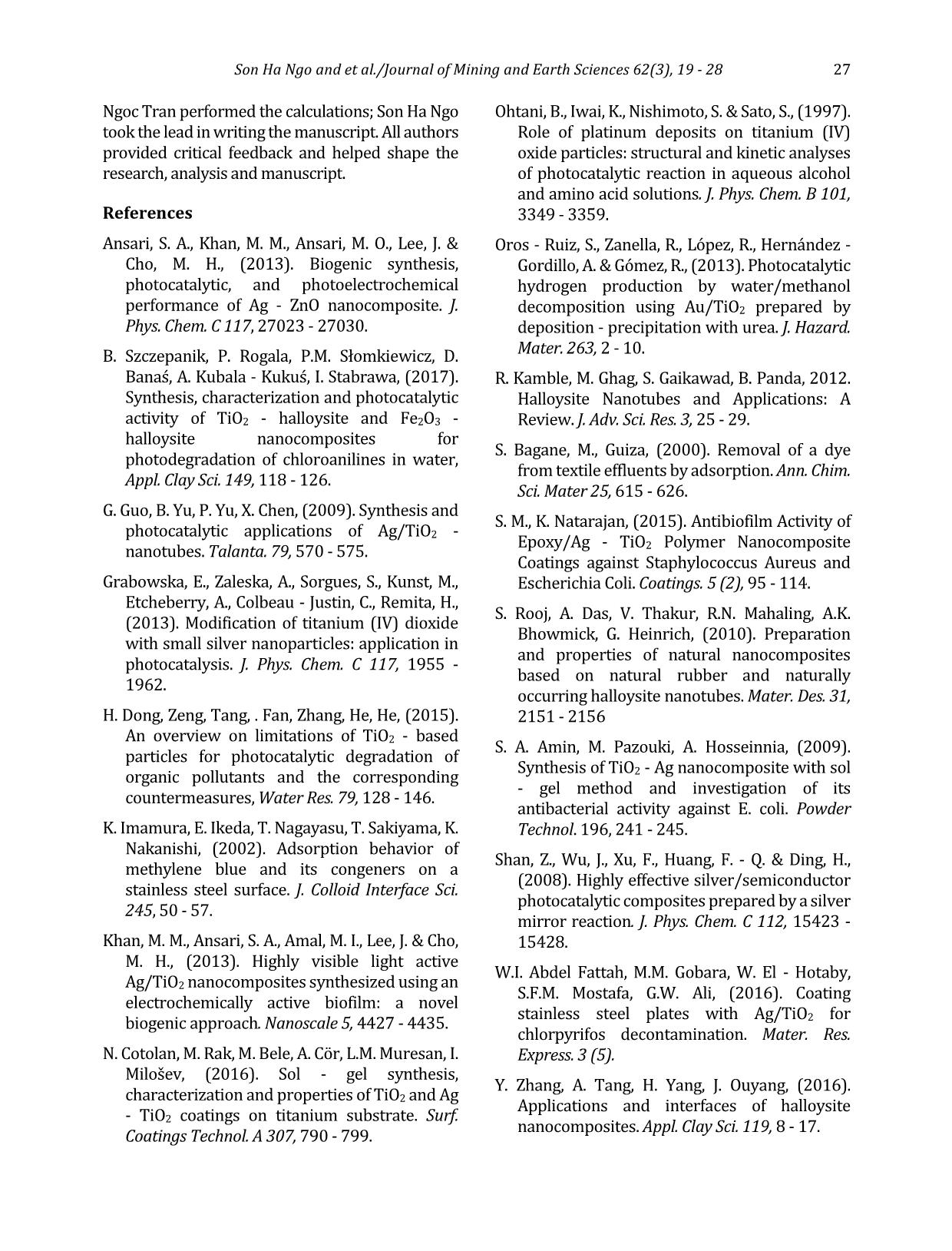
Trang 9
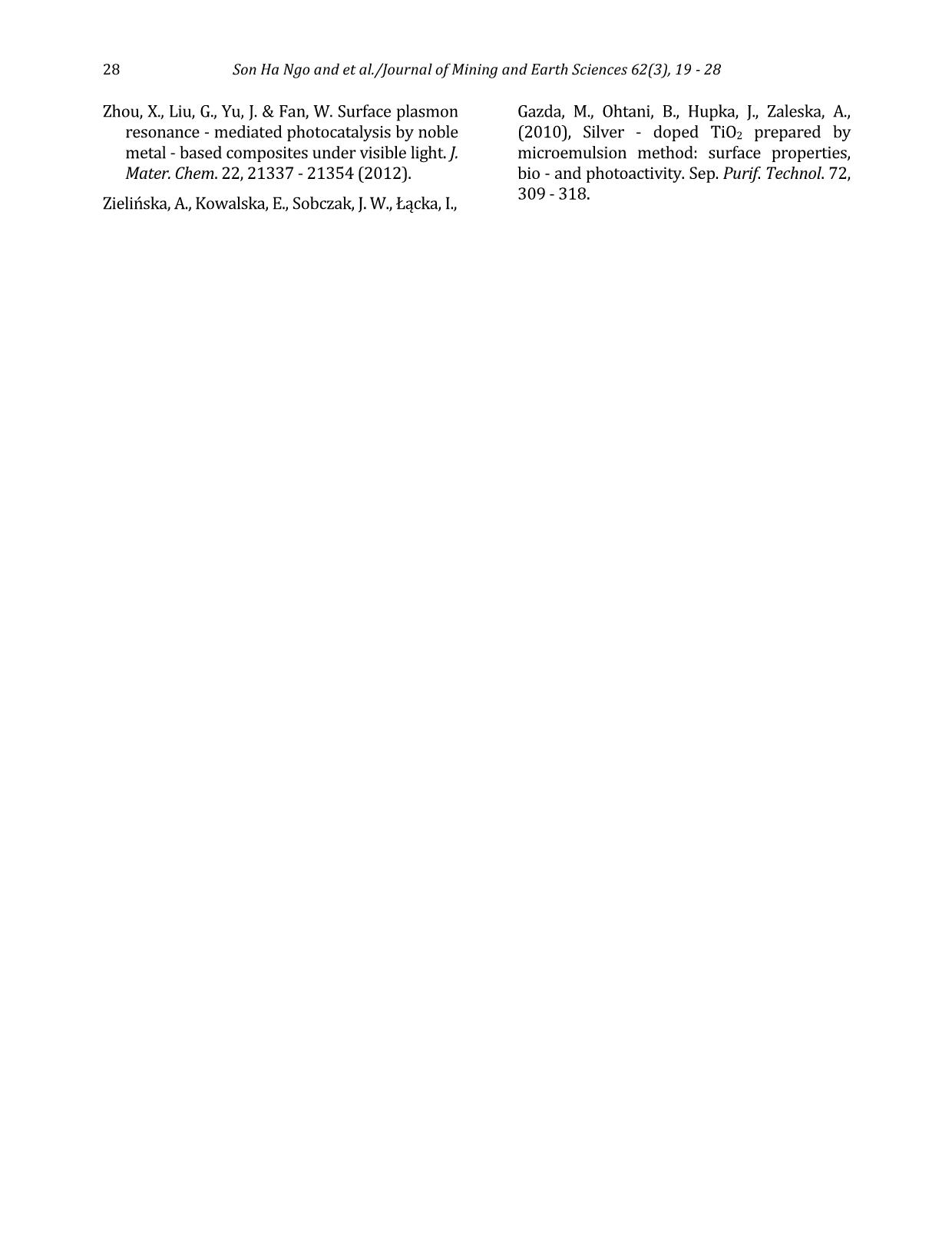
Trang 10
Tóm tắt nội dung tài liệu: Highly photocatalytic activity of natural halloysite - Based material for the treatment of dyes in wastewater
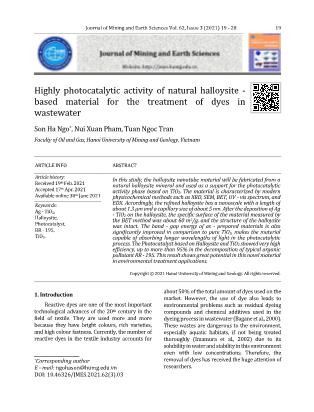
specific surface area and pore volume as well as pore diameter of HNT, the BET nitrogen adsorption - desorption isotherm at 77.3 K according was carried out for halloysite nanotube material samples. The curves are exhibited in Figure 3, showing that the adsorption - desorption isothermal curve of the HNT is of type IV and has a hysteresis curve H3 corresponding to the mesoporous material according to IUPAC classification. The pore size distribution curve illustrates that the mean pore diameter of HNT was 4.8 nm. Typical parameters of HNT obtained from BET measurement results: - Specific surface area (SBET): 28.0186 m2/g - Total pore volume (Vpore): 0.137840 cm3/g - Pore diameter (DBJH): 21.8489 nm. 3.1.4. Characterization of Ag - TiO2/HNT The results of XRD, UV - vis solid, EDX and SEM of Ag - TiO2/HNT materials are exhibited in Figures 4÷7. X - ray spectrum of Ag - TiO2/HNT material (Figure 4) still confirms the existence of the characteristic peaks of TiO2, which proves that there is no change in the structure of TiO2 material. Figure 3. Nitrogen adsorption - desorption isotherm at 77.3 K of HNT. Figure 4. X - ray (XRD) spectrum of different catalysts. Figure 5. Graph of [F(R)hν]2 as a function of hν for Ebg calculation. Figure 6. EDX spectrum of Ag - TiO2/HNT. 24 Son Ha Ngo and et al./Journal of Mining and Earth Sciences 62(3), 19 - 28 There are additional peaks which appear at 2θ = 38.1 (111) and 64.4 (220) and weaker intensity peak at 2θ = 44.3 (200) characterizing the presence of metallic silver (JCPDS 65 - 2871). The obtained results demonstrate that the insertion of TiO2 and Ag - TiO2 on the HNT support causes no effect on the halloysite nanotube structure. The band - gap energy Eg of the photocatalytic materials TiO2, Ag - TiO2, TiO2 - HNT, Ag - TiO2/HNT were 3.2 eV, 2.8 eV, 3.0 eV, and 2.6 eV, respectively that were indicated in Figure 5. Those values appear to verify that the combination of HNT and TiO2 has adjusted the band - gap of TiO2 3.2÷3 eV. Furthermore, the addition of Ag onto the surface of TiO2 has drastically reduced the band - gap of TiO2 to 2.8 eV that could consequently lead to a significant influence on the photocatalytic properties of TiO2. Thereby, the excited zone of Ag - TiO2/HNT shifted considerably from the ultraviolet (UV) to the visible (Vis) region, and the material has a narrower band - gap (2.6 eV) compared to pure TiO2 (3.2 eV) Also, the X - ray energy dispersive spectrum of Ag - TiO2/HNT in the binding energy range of 0÷10 keV in Figure 6 points out the corresponding signals of the elements that make up the Ag - TiO2/HNT catalyst and no different elements appear. Additionally, the mass percentage of the elements are quite compatible with the theoretical calculation used in the first step to synthesize Ag - TiO2/HNT. SEM, TEM images (Figure 7) presents that the implantation of Ag - TiO2 onto halloysite nanotubes did not break the halloysite's tube structure. The images show the uniform dispersion of the Ag - TiO2 nanoparticles on halloysite (HNT) nanotubes and the Ag nanoparticles that are tightly attached on the surface of the TiO2 nanorods to form Ag - TiO2 nanorods with an average size is about 10÷20 nm. As can be described in Figure 8 and Table 1, Ag - TiO2/HNT has twice as much specific surface area and significantly increased pore volume as the HNT support. It could be explained that Ag - TiO2 also has its own specific surface area and has its porous system. As a result, the presence of Ag - TiO2 on the inner and outer surface of halloysite nanotubes could contribute to the number of pores representing for the higher surface area as well as the total pore volume. The higher pore volume of Ag - TiO2/HNT could guarantee the better performance of this catalyst in terms of pollutants Figure 7. SEM (a and b), TEM (c and d) images of Ag - TiO2/HNT. Son Ha Ngo and et al./Journal of Mining and Earth Sciences 62(3), 19 - 28 25 adsorption. Based on the evidence given by various characterization techniques, it could be concluded that Ag - TiO2 was successfully grafted on the surface of HNT. Moreover, Ag - TiO2 particles in nano size, which were distributed evenly on the surface, could bring efficient catalytic activity to the as - prepared nanocomposite. Parameter Value Specific surface area (SBET - m2/ g) 57.3668 Pore diameter (DBJH - nm) 10.6906 Pore volume (Vpore - cm3/ g) 0.163701 Micropore volume (cm3/ g) 0.000193 3.2. Photocatalytic activity of Ag - TiO2/HNT 3.2.1. Comparison of photocatalytic activity of synthesized materials The photocatalytic decomposition of RR - 195 was performed at room temperature with the following conditions: the initial concentration of RR - 195 in water was 50 ppm, then 1.5 ml of H2O2 was added with 0.05 g solid catalyst. Next, the mixture was stirred for 3 hours to reach equilibrium adsorption. After that, the mixture was irradiated with UV light for the next 3 hours. The results of the catalytic activity evaluation are presented in Figure 9. The results pointed out that the Ag - TiO2/HNT synthesized by the direct method has the highest photocatalytic activity. This can be elucidated by the existence of Ag - TiO2 on the surface of halloysite reduced the band - gap energy of TiO2 (2.6 eV). In addition, Ago can be a center of charge transition and has high ability to trap electrons to prevent recombination of photo - generated electrons and holes on TiO2 surface. At the same time, silver also has a plasmon resonance effect resulting in the more OH radical generation and increased photocatalytic efficiency. 3.2.2. Impact of H2O2 concentration on the conversion of RR - 195 H2O2 has an important impact on the photocatalytic degradation of pollutants. The effect of H2O2 in RR - 195 was investigated, and the results are presented in Figure 10. As can be observed in Figure 10, when using 2 ml of H2O2 with the presence of Ag - TiO2/HNT catalyst under UV irradiation, the conversion of RR - 195 was 79% in a period of 360 minutes. When increasing the amount of H2O2 to 4ml, the mineralization of RR - 195 after 360 minutes did not occur faster but tended to decrease (76%). In addition, if the amount of H2O2 is reduced to 1ml and 1.5 ml, the rate as well as the decomposition efficiency RR - 195 decreased also standing at 66% and 76%, respectively. This is because more •OH radicals generated from H2O2 promote the reaction leading to increased decomposition rate and efficiency. However, when the amount of H2O2 in the solution is too high or too low will reduce the free radicals •OH occurs according to the equation (Szczepanik Figure 8. Nitrogen adsorption - desorption isotherm at 77.3K of Ag - TiO2/HNT catalyst. Table 1. Parameters of Ag - TiO2 - HNT obtained from BET method. Figure 9. Conversion of RR - 195 in photocatalytic degradation process using different catalysts. 26 Son Ha Ngo and et al./Journal of Mining and Earth Sciences 62(3), 19 - 28 et al., 2017; Abdel Fattah et al., 2016; Natarajan et al., 2015): H2O2 + •OH •HO2 + H2O •HO2 + •OH O2 + H2O (2) In addition, a high amount of H2O2 also results in the saturation of the active sites of the catalyst, thereby diminishing the reaction rate. For that reason, 1.5 ml of H2O2 was chosen to be applied to all remaining research processes to best assess the catalytic activity of the catalyst materials. 3.2.3. Influence of catalyst weight The influence of the catalyst content on the conversion of dye RR - 195 on Ag - TiO2/HNT catalyst was investigated. The results are shown in Figure 11. The results showed that after 360 minutes of UV irradiation using 0.15 g of Ag - TiO2/HNT, the efficiency in RR - 195 degradation was 96%. This value is considerably higher than the ones obtained when 0.05g and 0.1g catalyst was applied, which only reached the conversion of 66% and 90%, respectively. This can be explained as follows: when the amount of catalyst increases, the amount of catalytic activity sites increases, causing the diffusion rate of the anions RR - 195 to the active sites on the surface, leading to an increase the number of 96% shows the almost complete decomposition of contaminants under normal conditions. 4. Conclusions This study has obtained some remarkable results with a relatively new photocatalytic material system based on the halloysite natural mineral. Specifically, purified halloysite (HNT) nanotubes have been processed from raw halloysite sources in kaolin mines in a specific surface area of 28.0186 m2/g with an average length of 1.3 μm and average diameter of 130 nm. Ag - TiO2/HNT photocatalytic material was then synthesized by the direct method. The data showed that the doping of Ag significantly narrowed the band - gap energy of pure TiO2. Thus, the material could be easily excited in the visible region, which makes it more efficient in the photocatalytic process. In addition, the specific area and pore volume of Ag - TiO2/HNT were significantly improved in comparison with HNT and TiO2. The photocatalytic activity evaluation indicated that the Ag - TiO2/HNT had superior efficiency compared to the other catalysts namely TiO2; Ag - TiO2; TiO2/HNT thanks to the presence of Ag and the better distribution of active phase on HNT support. As a result, Ag - TiO2/HNT could almost completely decomposed RR - 195 at room temperature and neutral pH environment. This suggests that Ag - TiO2/HNT material could offer extreme potential in the treatment of polluted wastewater. Author contributions Son Ha Ngo and Nui Xuan Pham conceived and planned the experiments; Tuan Ngoc Tran carried out the experiments; Son Ha Ngo and Tuan Ngoc Tran contributed to sample preparation. Son Ha Ngo and Nui Xuan Pham contributed to the interpretation of the results; Son Ha Ngo and Tuan Figure 10. The conversion of RR - 195 when using Ag - TiO2/HNT at different H2O2 concentrations. Figure 11. Influence of catalyst weight in the degradation process of RR - 195. Son Ha Ngo and et al./Journal of Mining and Earth Sciences 62(3), 19 - 28 27 Ngoc Tran performed the calculations; Son Ha Ngo took the lead in writing the manuscript. All authors provided critical feedback and helped shape the research, analysis and manuscript. References Ansari, S. A., Khan, M. M., Ansari, M. O., Lee, J. & Cho, M. H., (2013). Biogenic synthesis, photocatalytic, and photoelectrochemical performance of Ag - ZnO nanocomposite. J. Phys. Chem. C 117, 27023 - 27030. B. Szczepanik, P. Rogala, P.M. Słomkiewicz, D. Banaś, A. Kubala - Kukuś, I. Stabrawa, (2017). Synthesis, characterization and photocatalytic activity of TiO2 - halloysite and Fe2O3 - halloysite nanocomposites for photodegradation of chloroanilines in water, Appl. Clay Sci. 149, 118 - 126. G. Guo, B. Yu, P. Yu, X. Chen, (2009). Synthesis and photocatalytic applications of Ag/TiO2 - nanotubes. Talanta. 79, 570 - 575. Grabowska, E., Zaleska, A., Sorgues, S., Kunst, M., Etcheberry, A., Colbeau - Justin, C., Remita, H., (2013). Modification of titanium (IV) dioxide with small silver nanoparticles: application in photocatalysis. J. Phys. Chem. C 117, 1955 - 1962. H. Dong, Zeng, Tang, . Fan, Zhang, He, He, (2015). An overview on limitations of TiO2 - based particles for photocatalytic degradation of organic pollutants and the corresponding countermeasures, Water Res. 79, 128 - 146. K. Imamura, E. Ikeda, T. Nagayasu, T. Sakiyama, K. Nakanishi, (2002). Adsorption behavior of methylene blue and its congeners on a stainless steel surface. J. Colloid Interface Sci. 245, 50 - 57. Khan, M. M., Ansari, S. A., Amal, M. I., Lee, J. & Cho, M. H., (2013). Highly visible light active Ag/TiO2 nanocomposites synthesized using an electrochemically active biofilm: a novel biogenic approach. Nanoscale 5, 4427 - 4435. N. Cotolan, M. Rak, M. Bele, A. Cör, L.M. Muresan, I. Milošev, (2016). Sol - gel synthesis, characterization and properties of TiO2 and Ag - TiO2 coatings on titanium substrate. Surf. Coatings Technol. A 307, 790 - 799. Ohtani, B., Iwai, K., Nishimoto, S. & Sato, S., (1997). Role of platinum deposits on titanium (IV) oxide particles: structural and kinetic analyses of photocatalytic reaction in aqueous alcohol and amino acid solutions. J. Phys. Chem. B 101, 3349 - 3359. Oros - Ruiz, S., Zanella, R., López, R., Hernández - Gordillo, A. & Gómez, R., (2013). Photocatalytic hydrogen production by water/methanol decomposition using Au/TiO2 prepared by deposition - precipitation with urea. J. Hazard. Mater. 263, 2 - 10. R. Kamble, M. Ghag, S. Gaikawad, B. Panda, 2012. Halloysite Nanotubes and Applications: A Review. J. Adv. Sci. Res. 3, 25 - 29. S. Bagane, M., Guiza, (2000). Removal of a dye from textile effluents by adsorption. Ann. Chim. Sci. Mater 25, 615 - 626. S. M., K. Natarajan, (2015). Antibiofilm Activity of Epoxy/Ag - TiO2 Polymer Nanocomposite Coatings against Staphylococcus Aureus and Escherichia Coli. Coatings. 5 (2), 95 - 114. S. Rooj, A. Das, V. Thakur, R.N. Mahaling, A.K. Bhowmick, G. Heinrich, (2010). Preparation and properties of natural nanocomposites based on natural rubber and naturally occurring halloysite nanotubes. Mater. Des. 31, 2151 - 2156 S. A. Amin, M. Pazouki, A. Hosseinnia, (2009). Synthesis of TiO2 - Ag nanocomposite with sol - gel method and investigation of its antibacterial activity against E. coli. Powder Technol. 196, 241 - 245. Shan, Z., Wu, J., Xu, F., Huang, F. - Q. & Ding, H., (2008). Highly effective silver/semiconductor photocatalytic composites prepared by a silver mirror reaction. J. Phys. Chem. C 112, 15423 - 15428. W.I. Abdel Fattah, M.M. Gobara, W. El - Hotaby, S.F.M. Mostafa, G.W. Ali, (2016). Coating stainless steel plates with Ag/TiO2 for chlorpyrifos decontamination. Mater. Res. Express. 3 (5). Y. Zhang, A. Tang, H. Yang, J. Ouyang, (2016). Applications and interfaces of halloysite nanocomposites. Appl. Clay Sci. 119, 8 - 17. 28 Son Ha Ngo and et al./Journal of Mining and Earth Sciences 62(3), 19 - 28 Zhou, X., Liu, G., Yu, J. & Fan, W. Surface plasmon resonance - mediated photocatalysis by noble metal - based composites under visible light. J. Mater. Chem. 22, 21337 - 21354 (2012). Zielińska, A., Kowalska, E., Sobczak, J. W., Łącka, I., Gazda, M., Ohtani, B., Hupka, J., Zaleska, A., (2010), Silver - doped TiO2 prepared by microemulsion method: surface properties, bio - and photoactivity. Sep. Purif. Technol. 72, 309 - 318.
File đính kèm:
highly_photocatalytic_activity_of_natural_halloysite_based_m.pdf