Dosimetric characteristics of 6 MV photons from TrueBeam STx medical linear accelerator: Simulation and experimental data
A TrueBeam STx is one of the most technologically advanced linear accelerators for
radiotherapy and radiosurgery. The Monte Carlo simulation widely used in many applications in
various fields such as nuclear physics, astrophysics, particle physics, and medicine. The
Geant4/GATE Monte Carlo toolkit is developed for the simulation in imaging diagnostics, nuclear
medicine, radiotherapy, and radiation biology to more accurately predict beam radiation dosimetry. In
this work, we present the simulation results of the dosimetric characteristics of a 6 MV photon beam
of TrueBeam STx medical LINAC using Monte Carlo Geant4/GATE. The percentage depth dose
(PDD), central axis depth dose (Profile) have been simulated and compared with those measured in a
water phantom for field sizes 10×10 cm2 via the gamma-index method. These results will permit to
check calculation data given by the treatment planning system.
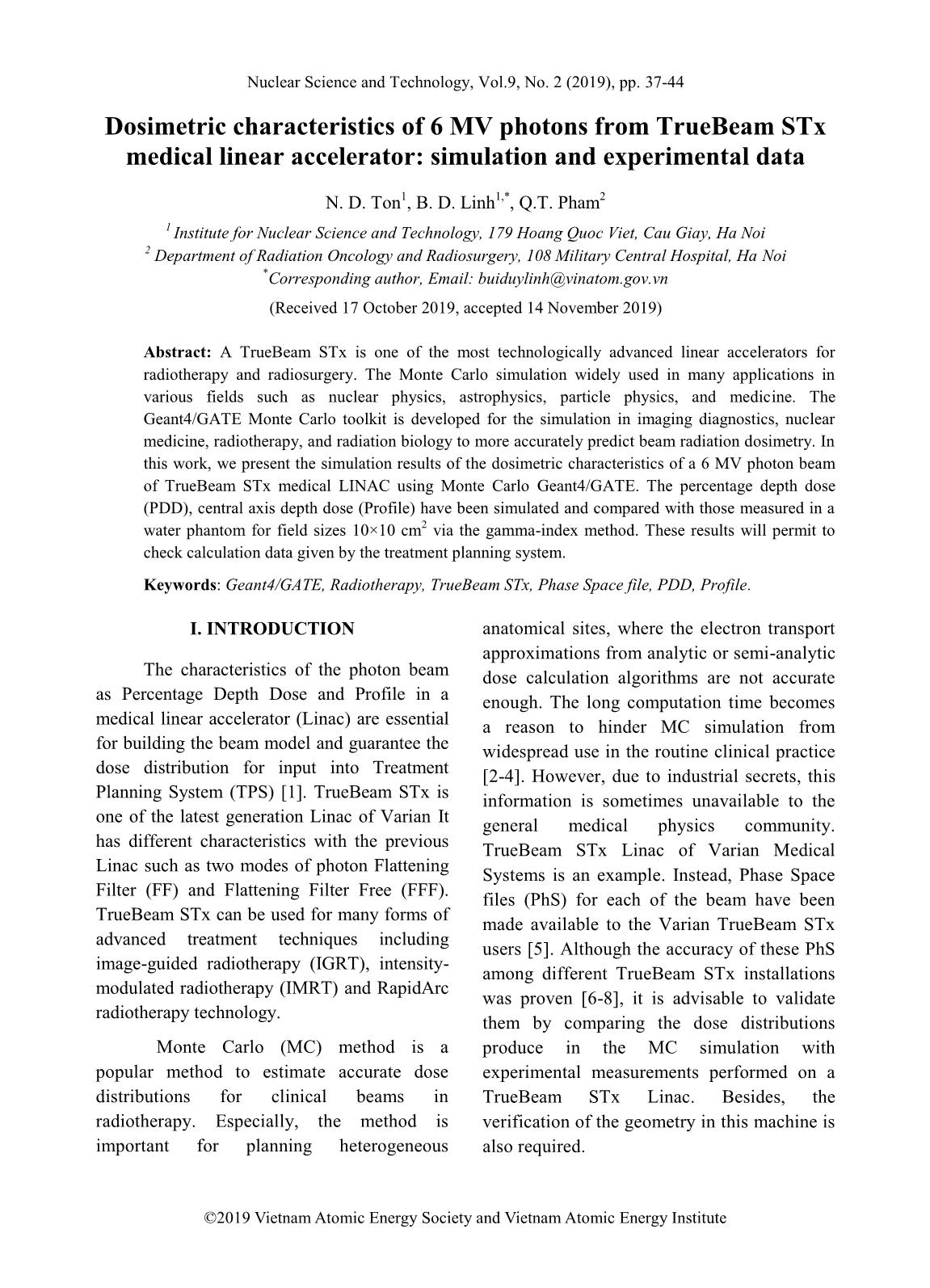
Trang 1
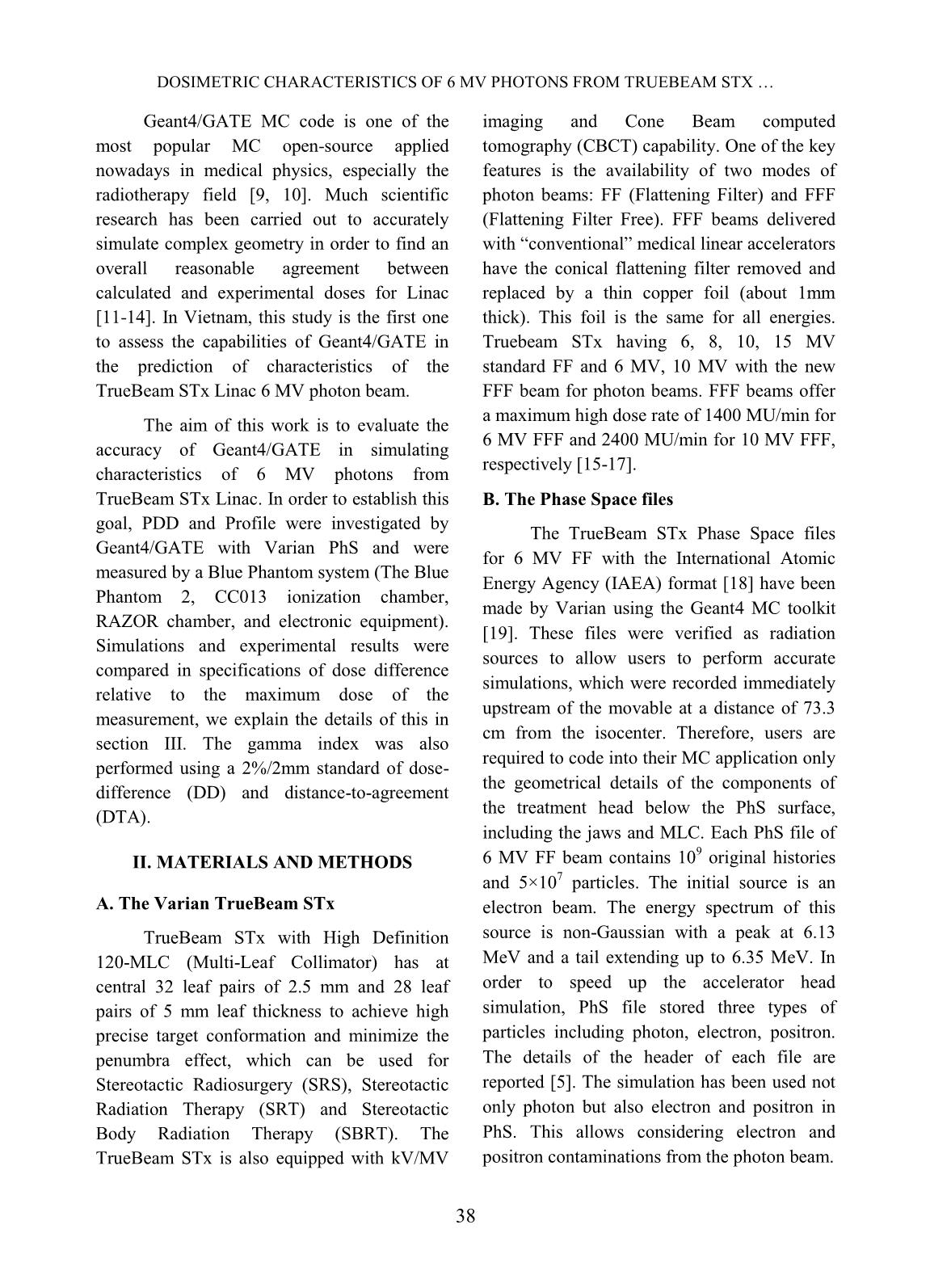
Trang 2
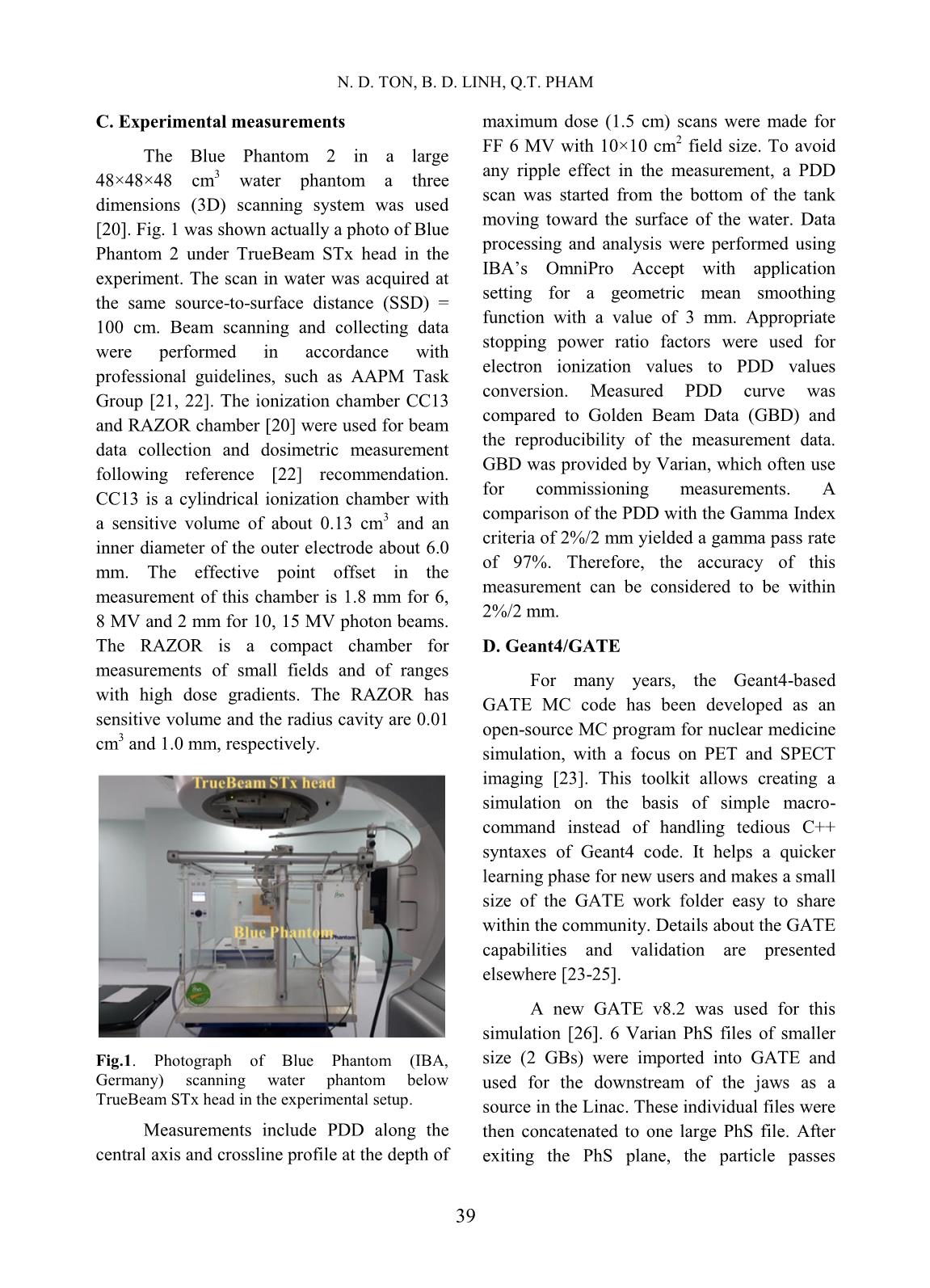
Trang 3
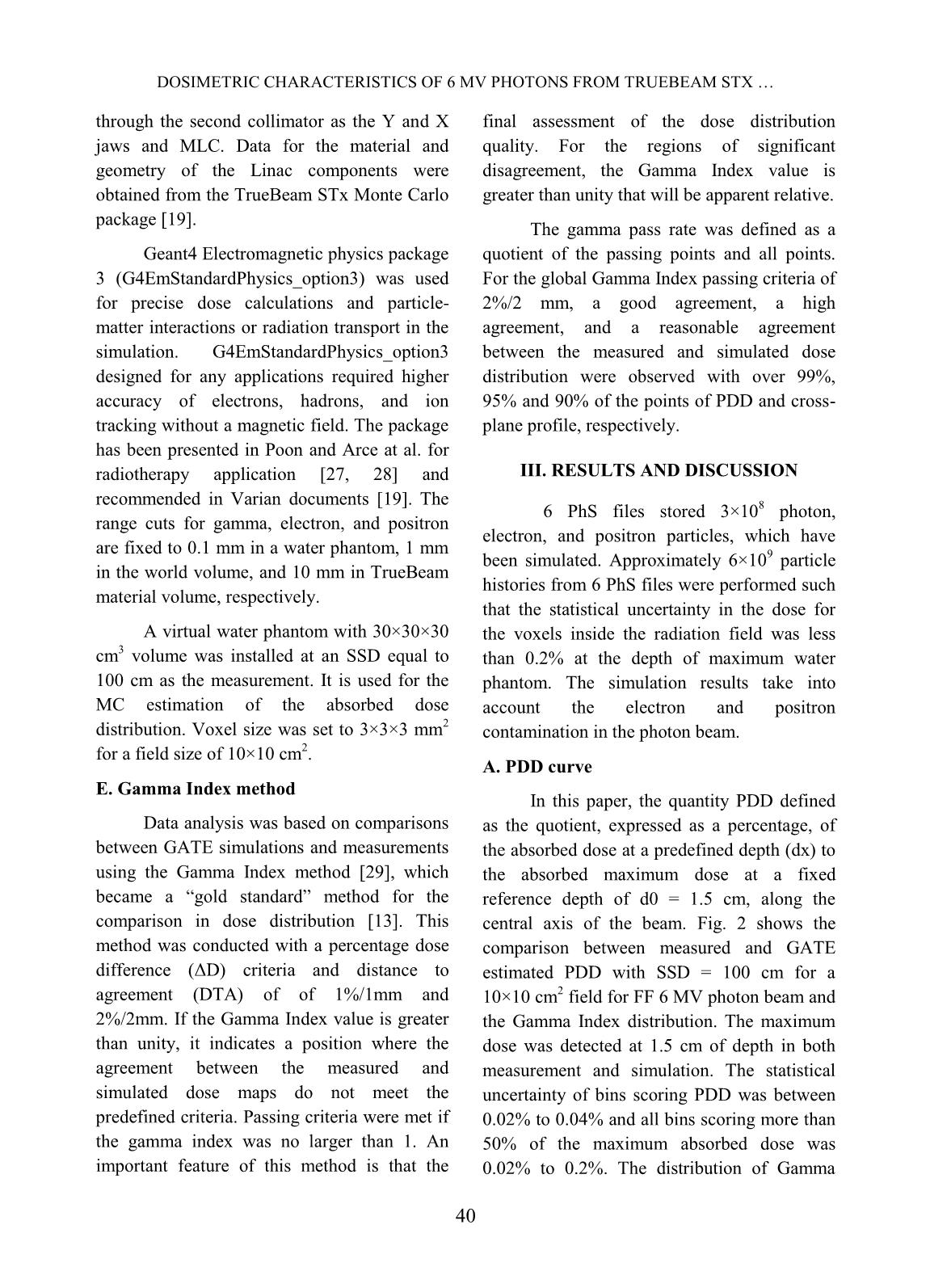
Trang 4
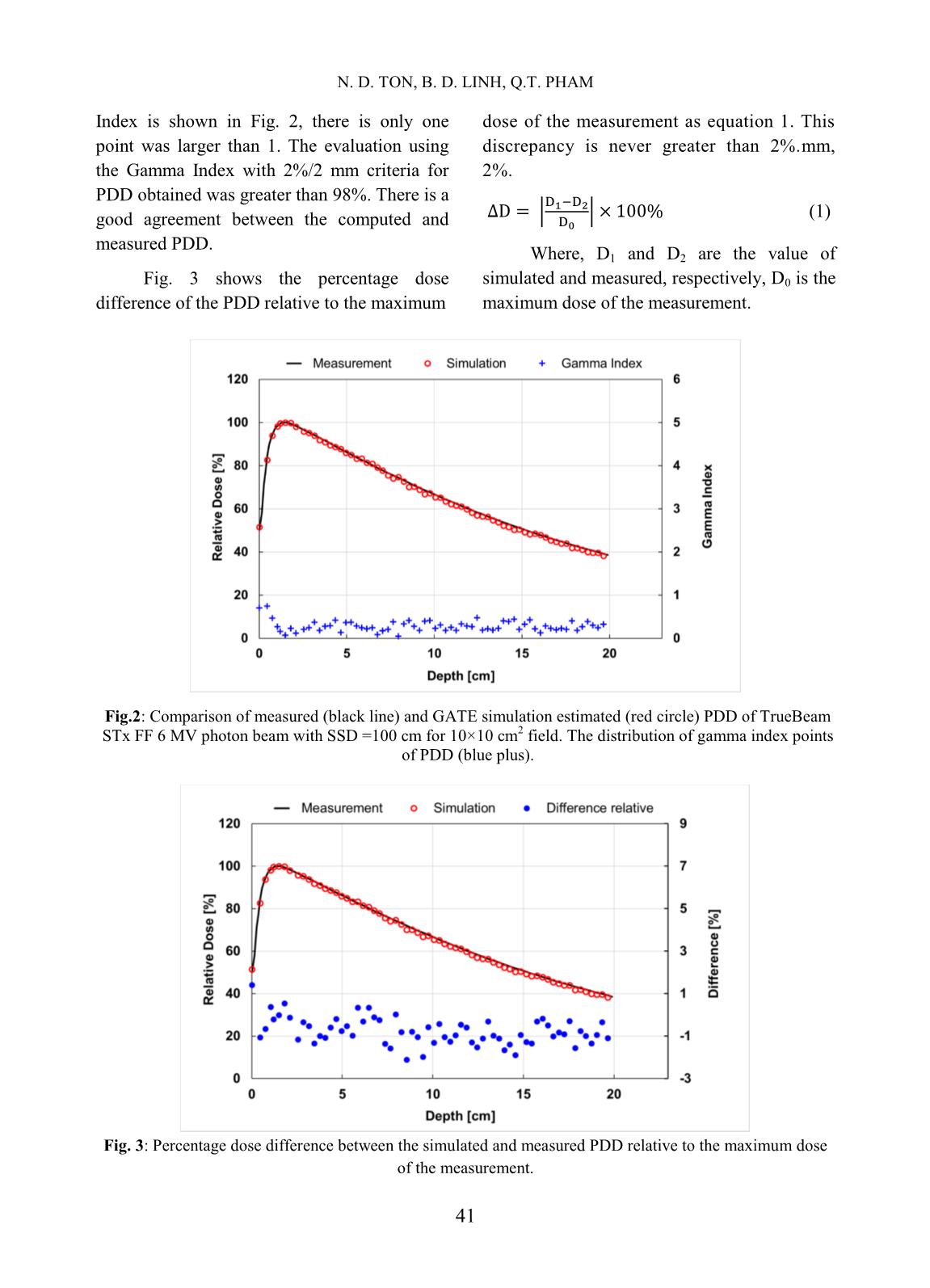
Trang 5
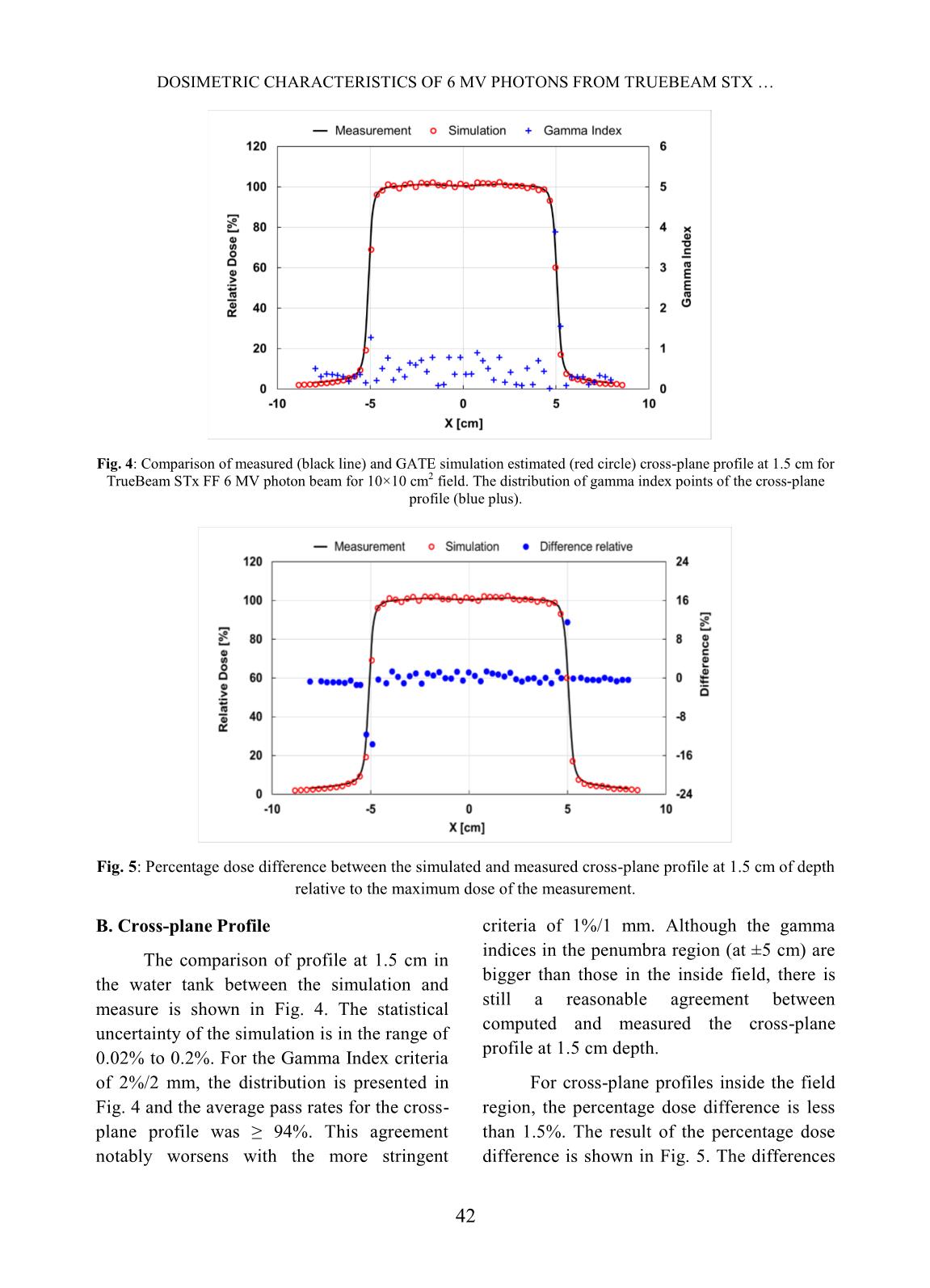
Trang 6
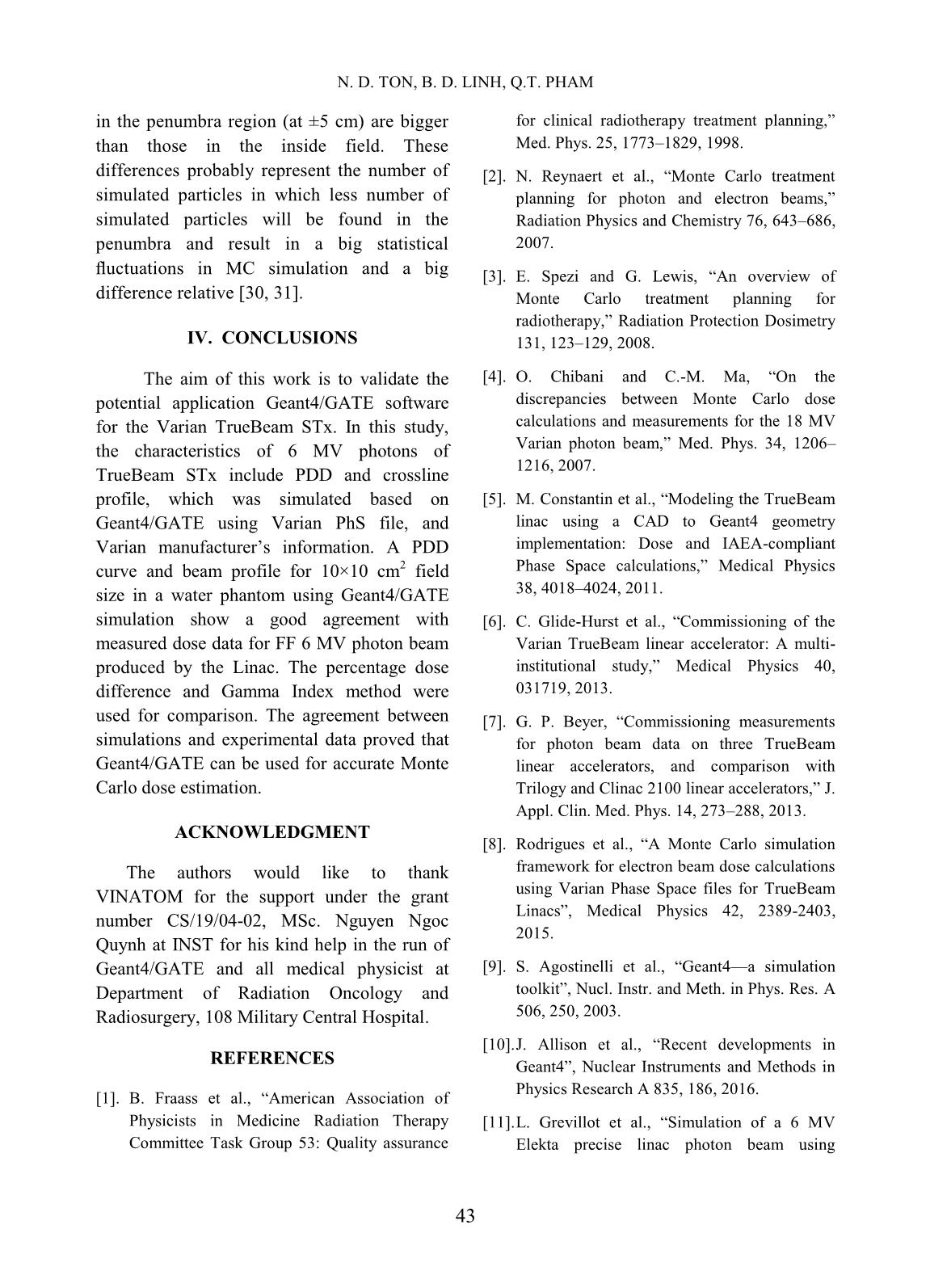
Trang 7
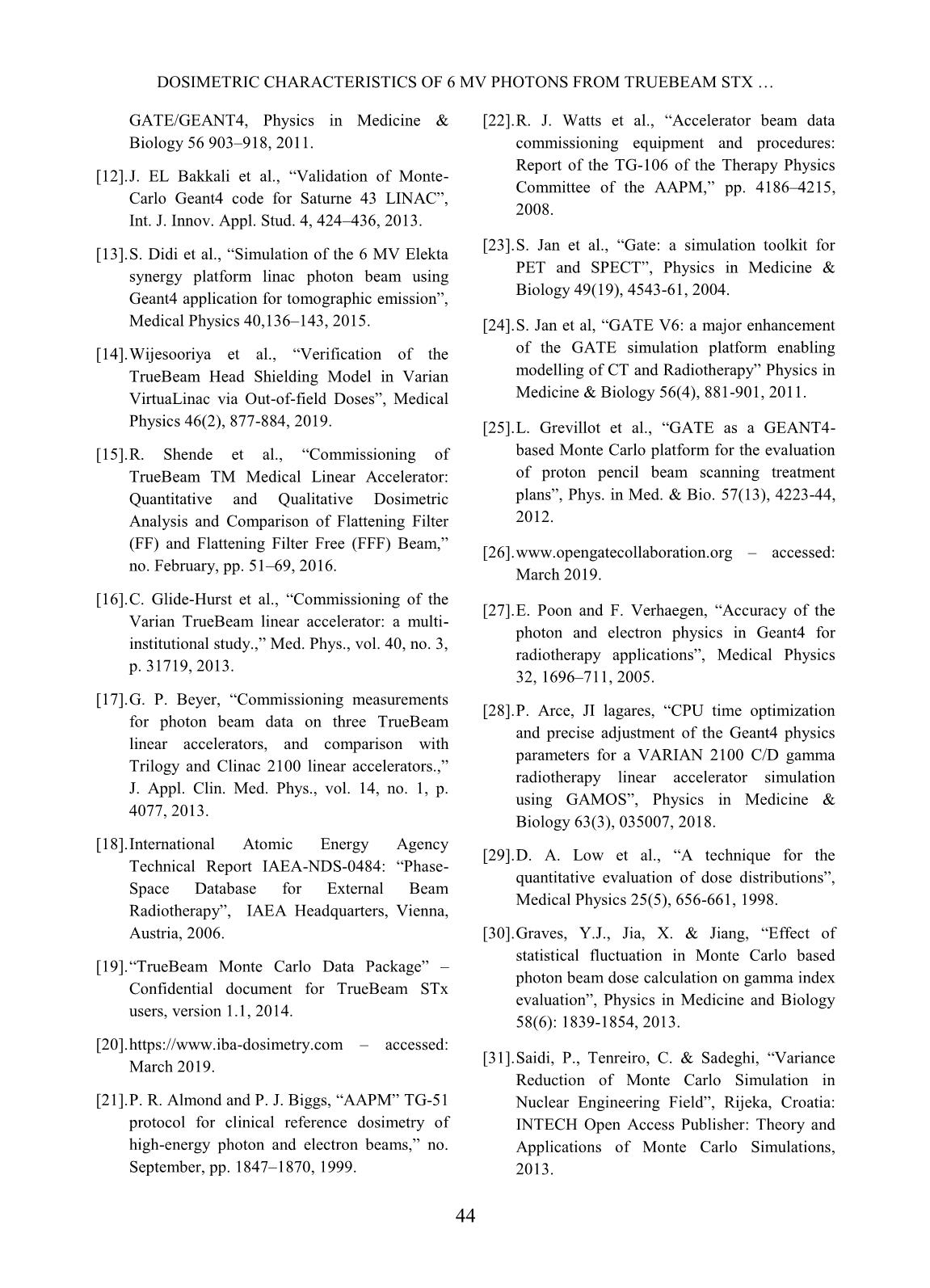
Trang 8
Tóm tắt nội dung tài liệu: Dosimetric characteristics of 6 MV photons from TrueBeam STx medical linear accelerator: Simulation and experimental data
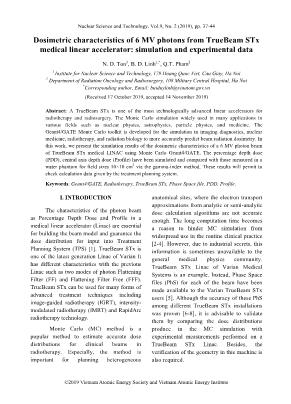
s of small fields and of ranges with high dose gradients. The RAZOR has sensitive volume and the radius cavity are 0.01 cm 3 and 1.0 mm, respectively. Fig.1. Photograph of Blue Phantom (IBA, Germany) scanning water phantom below TrueBeam STx head in the experimental setup. Measurements include PDD along the central axis and crossline profile at the depth of maximum dose (1.5 cm) scans were made for FF 6 MV with 10×10 cm 2 field size. To avoid any ripple effect in the measurement, a PDD scan was started from the bottom of the tank moving toward the surface of the water. Data processing and analysis were performed using IBA’s OmniPro Accept with application setting for a geometric mean smoothing function with a value of 3 mm. Appropriate stopping power ratio factors were used for electron ionization values to PDD values conversion. Measured PDD curve was compared to Golden Beam Data (GBD) and the reproducibility of the measurement data. GBD was provided by Varian, which often use for commissioning measurements. A comparison of the PDD with the Gamma Index criteria of 2%/2 mm yielded a gamma pass rate of 97%. Therefore, the accuracy of this measurement can be considered to be within 2%/2 mm. D. Geant4/GATE For many years, the Geant4-based GATE MC code has been developed as an open-source MC program for nuclear medicine simulation, with a focus on PET and SPECT imaging [23]. This toolkit allows creating a simulation on the basis of simple macro- command instead of handling tedious C++ syntaxes of Geant4 code. It helps a quicker learning phase for new users and makes a small size of the GATE work folder easy to share within the community. Details about the GATE capabilities and validation are presented elsewhere [23-25]. A new GATE v8.2 was used for this simulation [26]. 6 Varian PhS files of smaller size (2 GBs) were imported into GATE and used for the downstream of the jaws as a source in the Linac. These individual files were then concatenated to one large PhS file. After exiting the PhS plane, the particle passes DOSIMETRIC CHARACTERISTICS OF 6 MV PHOTONS FROM TRUEBEAM STX 40 through the second collimator as the Y and X jaws and MLC. Data for the material and geometry of the Linac components were obtained from the TrueBeam STx Monte Carlo package [19]. Geant4 Electromagnetic physics package 3 (G4EmStandardPhysics_option3) was used for precise dose calculations and particle- matter interactions or radiation transport in the simulation. G4EmStandardPhysics_option3 designed for any applications required higher accuracy of electrons, hadrons, and ion tracking without a magnetic field. The package has been presented in Poon and Arce at al. for radiotherapy application [27, 28] and recommended in Varian documents [19]. The range cuts for gamma, electron, and positron are fixed to 0.1 mm in a water phantom, 1 mm in the world volume, and 10 mm in TrueBeam material volume, respectively. A virtual water phantom with 30×30×30 cm 3 volume was installed at an SSD equal to 100 cm as the measurement. It is used for the MC estimation of the absorbed dose distribution. Voxel size was set to 3×3×3 mm 2 for a field size of 10×10 cm 2 . E. Gamma Index method Data analysis was based on comparisons between GATE simulations and measurements using the Gamma Index method [29], which became a ―gold standard‖ method for the comparison in dose distribution [13]. This method was conducted with a percentage dose difference (ΔD) criteria and distance to agreement (DTA) of of 1%/1mm and 2%/2mm. If the Gamma Index value is greater than unity, it indicates a position where the agreement between the measured and simulated dose maps do not meet the predefined criteria. Passing criteria were met if the gamma index was no larger than 1. An important feature of this method is that the final assessment of the dose distribution quality. For the regions of significant disagreement, the Gamma Index value is greater than unity that will be apparent relative. The gamma pass rate was defined as a quotient of the passing points and all points. For the global Gamma Index passing criteria of 2%/2 mm, a good agreement, a high agreement, and a reasonable agreement between the measured and simulated dose distribution were observed with over 99%, 95% and 90% of the points of PDD and cross- plane profile, respectively. III. RESULTS AND DISCUSSION 6 PhS files stored 3×10 8 photon, electron, and positron particles, which have been simulated. Approximately 6×10 9 particle histories from 6 PhS files were performed such that the statistical uncertainty in the dose for the voxels inside the radiation field was less than 0.2% at the depth of maximum water phantom. The simulation results take into account the electron and positron contamination in the photon beam. A. PDD curve In this paper, the quantity PDD defined as the quotient, expressed as a percentage, of the absorbed dose at a predefined depth (dx) to the absorbed maximum dose at a fixed reference depth of d0 = 1.5 cm, along the central axis of the beam. Fig. 2 shows the comparison between measured and GATE estimated PDD with SSD = 100 cm for a 10×10 cm 2 field for FF 6 MV photon beam and the Gamma Index distribution. The maximum dose was detected at 1.5 cm of depth in both measurement and simulation. The statistical uncertainty of bins scoring PDD was between 0.02% to 0.04% and all bins scoring more than 50% of the maximum absorbed dose was 0.02% to 0.2%. The distribution of Gamma N. D. TON, B. D. LINH, Q.T. PHAM 41 Index is shown in Fig. 2, there is only one point was larger than 1. The evaluation using the Gamma Index with 2%/2 mm criteria for PDD obtained was greater than 98%. There is a good agreement between the computed and measured PDD. Fig. 3 shows the percentage dose difference of the PDD relative to the maximum dose of the measurement as equation 1. This discrepancy is never greater than 2%.mm, 2%. | | (1) Where, D1 and D2 are the value of simulated and measured, respectively, D0 is the maximum dose of the measurement. Fig.2: Comparison of measured (black line) and GATE simulation estimated (red circle) PDD of TrueBeam STx FF 6 MV photon beam with SSD =100 cm for 10×10 cm 2 field. The distribution of gamma index points of PDD (blue plus). Fig. 3: Percentage dose difference between the simulated and measured PDD relative to the maximum dose of the measurement. DOSIMETRIC CHARACTERISTICS OF 6 MV PHOTONS FROM TRUEBEAM STX 42 Fig. 4: Comparison of measured (black line) and GATE simulation estimated (red circle) cross-plane profile at 1.5 cm for TrueBeam STx FF 6 MV photon beam for 10×10 cm2 field. The distribution of gamma index points of the cross-plane profile (blue plus). Fig. 5: Percentage dose difference between the simulated and measured cross-plane profile at 1.5 cm of depth relative to the maximum dose of the measurement. B. Cross-plane Profile The comparison of profile at 1.5 cm in the water tank between the simulation and measure is shown in Fig. 4. The statistical uncertainty of the simulation is in the range of 0.02% to 0.2%. For the Gamma Index criteria of 2%/2 mm, the distribution is presented in Fig. 4 and the average pass rates for the cross- plane profile was ≥ 94%. This agreement notably worsens with the more stringent criteria of 1%/1 mm. Although the gamma indices in the penumbra region (at ±5 cm) are bigger than those in the inside field, there is still a reasonable agreement between computed and measured the cross-plane profile at 1.5 cm depth. For cross-plane profiles inside the field region, the percentage dose difference is less than 1.5%. The result of the percentage dose difference is shown in Fig. 5. The differences N. D. TON, B. D. LINH, Q.T. PHAM 43 in the penumbra region (at ±5 cm) are bigger than those in the inside field. These differences probably represent the number of simulated particles in which less number of simulated particles will be found in the penumbra and result in a big statistical fluctuations in MC simulation and a big difference relative [30, 31]. IV. CONCLUSIONS The aim of this work is to validate the potential application Geant4/GATE software for the Varian TrueBeam STx. In this study, the characteristics of 6 MV photons of TrueBeam STx include PDD and crossline profile, which was simulated based on Geant4/GATE using Varian PhS file, and Varian manufacturer’s information. A PDD curve and beam profile for 10×10 cm 2 field size in a water phantom using Geant4/GATE simulation show a good agreement with measured dose data for FF 6 MV photon beam produced by the Linac. The percentage dose difference and Gamma Index method were used for comparison. The agreement between simulations and experimental data proved that Geant4/GATE can be used for accurate Monte Carlo dose estimation. ACKNOWLEDGMENT The authors would like to thank VINATOM for the support under the grant number CS/19/04-02, MSc. Nguyen Ngoc Quynh at INST for his kind help in the run of Geant4/GATE and all medical physicist at Department of Radiation Oncology and Radiosurgery, 108 Military Central Hospital. REFERENCES [1]. B. Fraass et al., ―American Association of Physicists in Medicine Radiation Therapy Committee Task Group 53: Quality assurance for clinical radiotherapy treatment planning,‖ Med. Phys. 25, 1773–1829, 1998. [2]. N. Reynaert et al., ―Monte Carlo treatment planning for photon and electron beams,‖ Radiation Physics and Chemistry 76, 643–686, 2007. [3]. E. Spezi and G. Lewis, ―An overview of Monte Carlo treatment planning for radiotherapy,‖ Radiation Protection Dosimetry 131, 123–129, 2008. [4]. O. Chibani and C.-M. Ma, ―On the discrepancies between Monte Carlo dose calculations and measurements for the 18 MV Varian photon beam,‖ Med. Phys. 34, 1206– 1216, 2007. [5]. M. Constantin et al., ―Modeling the TrueBeam linac using a CAD to Geant4 geometry implementation: Dose and IAEA-compliant Phase Space calculations,‖ Medical Physics 38, 4018–4024, 2011. [6]. C. Glide-Hurst et al., ―Commissioning of the Varian TrueBeam linear accelerator: A multi- institutional study,‖ Medical Physics 40, 031719, 2013. [7]. G. P. Beyer, ―Commissioning measurements for photon beam data on three TrueBeam linear accelerators, and comparison with Trilogy and Clinac 2100 linear accelerators,‖ J. Appl. Clin. Med. Phys. 14, 273–288, 2013. [8]. Rodrigues et al., ―A Monte Carlo simulation framework for electron beam dose calculations using Varian Phase Space files for TrueBeam Linacs‖, Medical Physics 42, 2389-2403, 2015. [9]. S. Agostinelli et al., ―Geant4—a simulation toolkit‖, Nucl. Instr. and Meth. in Phys. Res. A 506, 250, 2003. [10]. J. Allison et al., ―Recent developments in Geant4‖, Nuclear Instruments and Methods in Physics Research A 835, 186, 2016. [11]. L. Grevillot et al., ―Simulation of a 6 MV Elekta precise linac photon beam using DOSIMETRIC CHARACTERISTICS OF 6 MV PHOTONS FROM TRUEBEAM STX 44 GATE/GEANT4, Physics in Medicine & Biology 56 903–918, 2011. [12]. J. EL Bakkali et al., ―Validation of Monte- Carlo Geant4 code for Saturne 43 LINAC‖, Int. J. Innov. Appl. Stud. 4, 424–436, 2013. [13]. S. Didi et al., ―Simulation of the 6 MV Elekta synergy platform linac photon beam using Geant4 application for tomographic emission‖, Medical Physics 40,136–143, 2015. [14]. Wijesooriya et al., ―Verification of the TrueBeam Head Shielding Model in Varian VirtuaLinac via Out-of-field Doses‖, Medical Physics 46(2), 877-884, 2019. [15]. R. Shende et al., ―Commissioning of TrueBeam TM Medical Linear Accelerator: Quantitative and Qualitative Dosimetric Analysis and Comparison of Flattening Filter (FF) and Flattening Filter Free (FFF) Beam,‖ no. February, pp. 51–69, 2016. [16]. C. Glide-Hurst et al., ―Commissioning of the Varian TrueBeam linear accelerator: a multi- institutional study.,‖ Med. Phys., vol. 40, no. 3, p. 31719, 2013. [17]. G. P. Beyer, ―Commissioning measurements for photon beam data on three TrueBeam linear accelerators, and comparison with Trilogy and Clinac 2100 linear accelerators.,‖ J. Appl. Clin. Med. Phys., vol. 14, no. 1, p. 4077, 2013. [18]. International Atomic Energy Agency Technical Report IAEA-NDS-0484: ―Phase- Space Database for External Beam Radiotherapy‖, IAEA Headquarters, Vienna, Austria, 2006. [19]. ―TrueBeam Monte Carlo Data Package‖ – Confidential document for TrueBeam STx users, version 1.1, 2014. [20]. https://www.iba-dosimetry.com – accessed: March 2019. [21]. P. R. Almond and P. J. Biggs, ―AAPM‖ TG-51 protocol for clinical reference dosimetry of high-energy photon and electron beams,‖ no. September, pp. 1847–1870, 1999. [22]. R. J. Watts et al., ―Accelerator beam data commissioning equipment and procedures: Report of the TG-106 of the Therapy Physics Committee of the AAPM,‖ pp. 4186–4215, 2008. [23]. S. Jan et al., ―Gate: a simulation toolkit for PET and SPECT‖, Physics in Medicine & Biology 49(19), 4543-61, 2004. [24]. S. Jan et al, ―GATE V6: a major enhancement of the GATE simulation platform enabling modelling of CT and Radiotherapy‖ Physics in Medicine & Biology 56(4), 881-901, 2011. [25]. L. Grevillot et al., ―GATE as a GEANT4- based Monte Carlo platform for the evaluation of proton pencil beam scanning treatment plans‖, Phys. in Med. & Bio. 57(13), 4223-44, 2012. [26]. www.opengatecollaboration.org – accessed: March 2019. [27]. E. Poon and F. Verhaegen, ―Accuracy of the photon and electron physics in Geant4 for radiotherapy applications‖, Medical Physics 32, 1696–711, 2005. [28]. P. Arce, JI lagares, ―CPU time optimization and precise adjustment of the Geant4 physics parameters for a VARIAN 2100 C/D gamma radiotherapy linear accelerator simulation using GAMOS‖, Physics in Medicine & Biology 63(3), 035007, 2018. [29]. D. A. Low et al., ―A technique for the quantitative evaluation of dose distributions‖, Medical Physics 25(5), 656-661, 1998. [30]. Graves, Y.J., Jia, X. & Jiang, ―Effect of statistical fluctuation in Monte Carlo based photon beam dose calculation on gamma index evaluation‖, Physics in Medicine and Biology 58(6): 1839-1854, 2013. [31]. Saidi, P., Tenreiro, C. & Sadeghi, ―Variance Reduction of Monte Carlo Simulation in Nuclear Engineering Field‖, Rijeka, Croatia: INTECH Open Access Publisher: Theory and Applications of Monte Carlo Simulations, 2013.
File đính kèm:
dosimetric_characteristics_of_6_mv_photons_from_truebeam_stx.pdf