Conceptual nuclear design of a 20 MW multipurpose research reactor
This paper presents some of studied results of a pre-feasibility project on a new research
reactor for Vietnam. In this work, two conceptual nuclear designs of 20 MW multi-purpose research
reactor have been done. The reference reactor is the light water cooled and heavy water reflected
open-tank-in-pool type reactor. The reactor model is based on the experiences from the operation and
utilization of the HANARO. Two fuel types, rod and flat plate, with dispersed U3Si2-Al fuel meat are
used in this study for comparison purpose. Analyses for the nuclear design parameters such as the
neutron flux, power distribution, reactivity coefficients, control rod worth, etc. have been done and the
equilibrium cores have been established to meet the requirements of nuclear safety and performance.
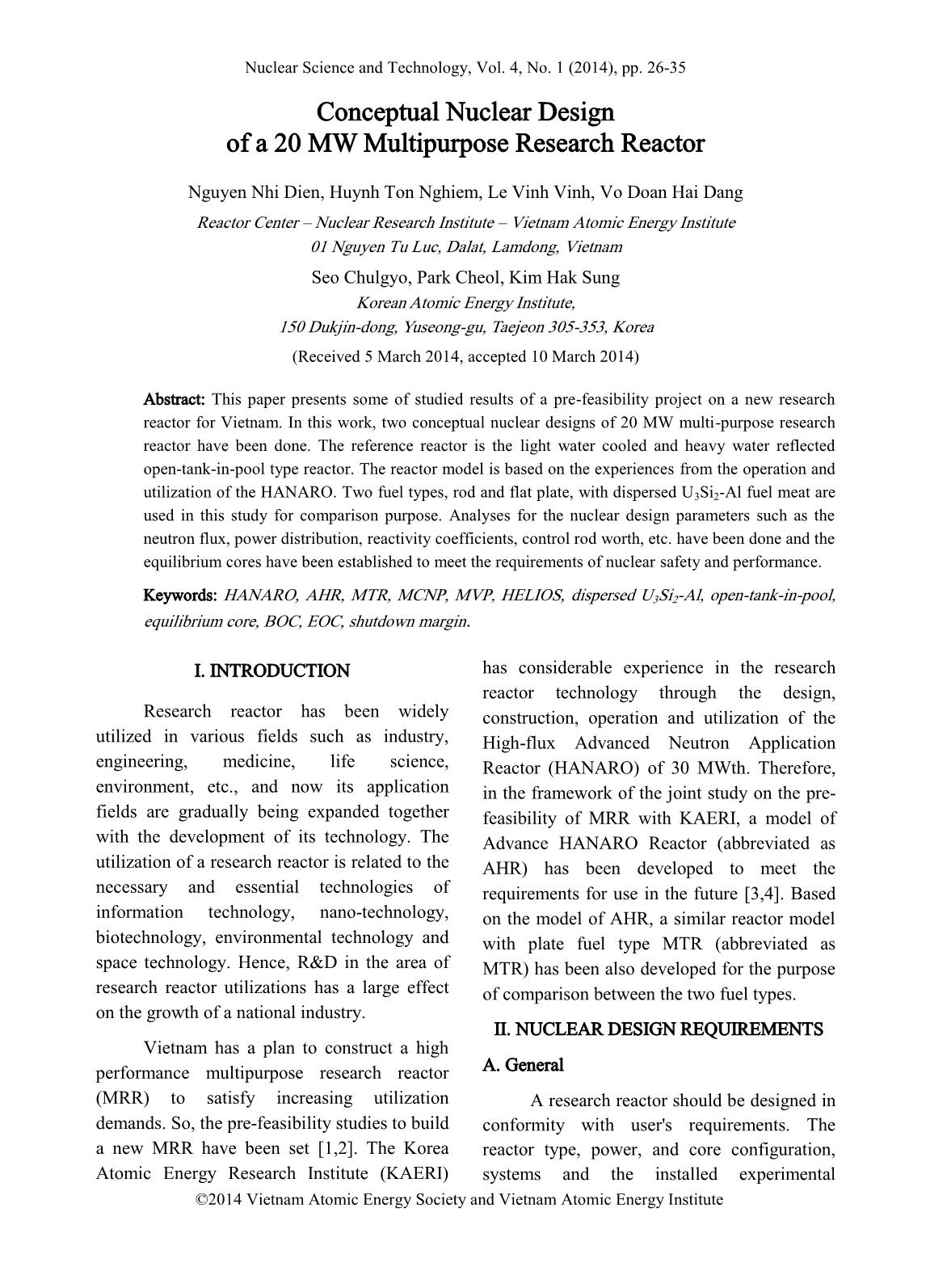
Trang 1
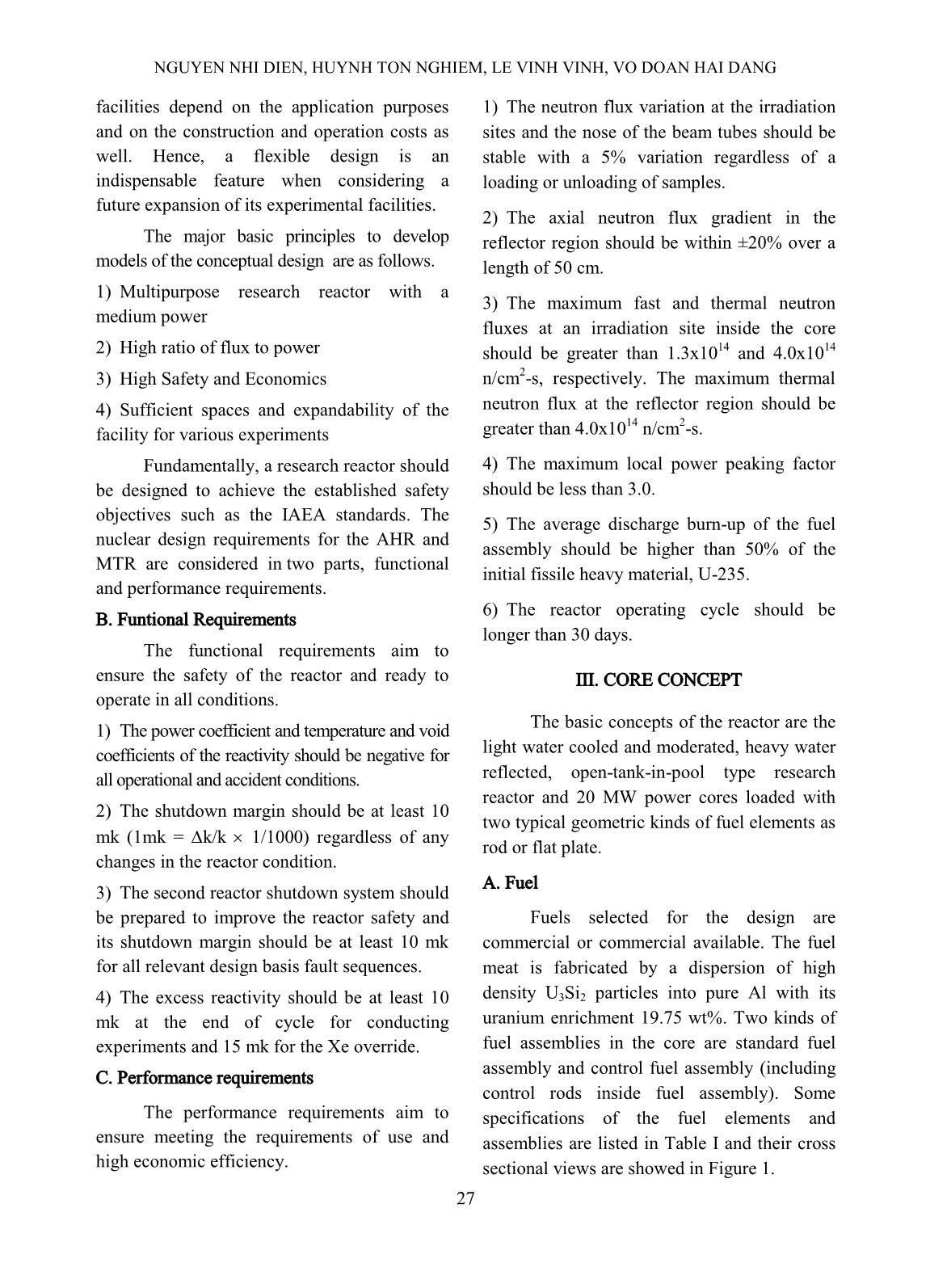
Trang 2
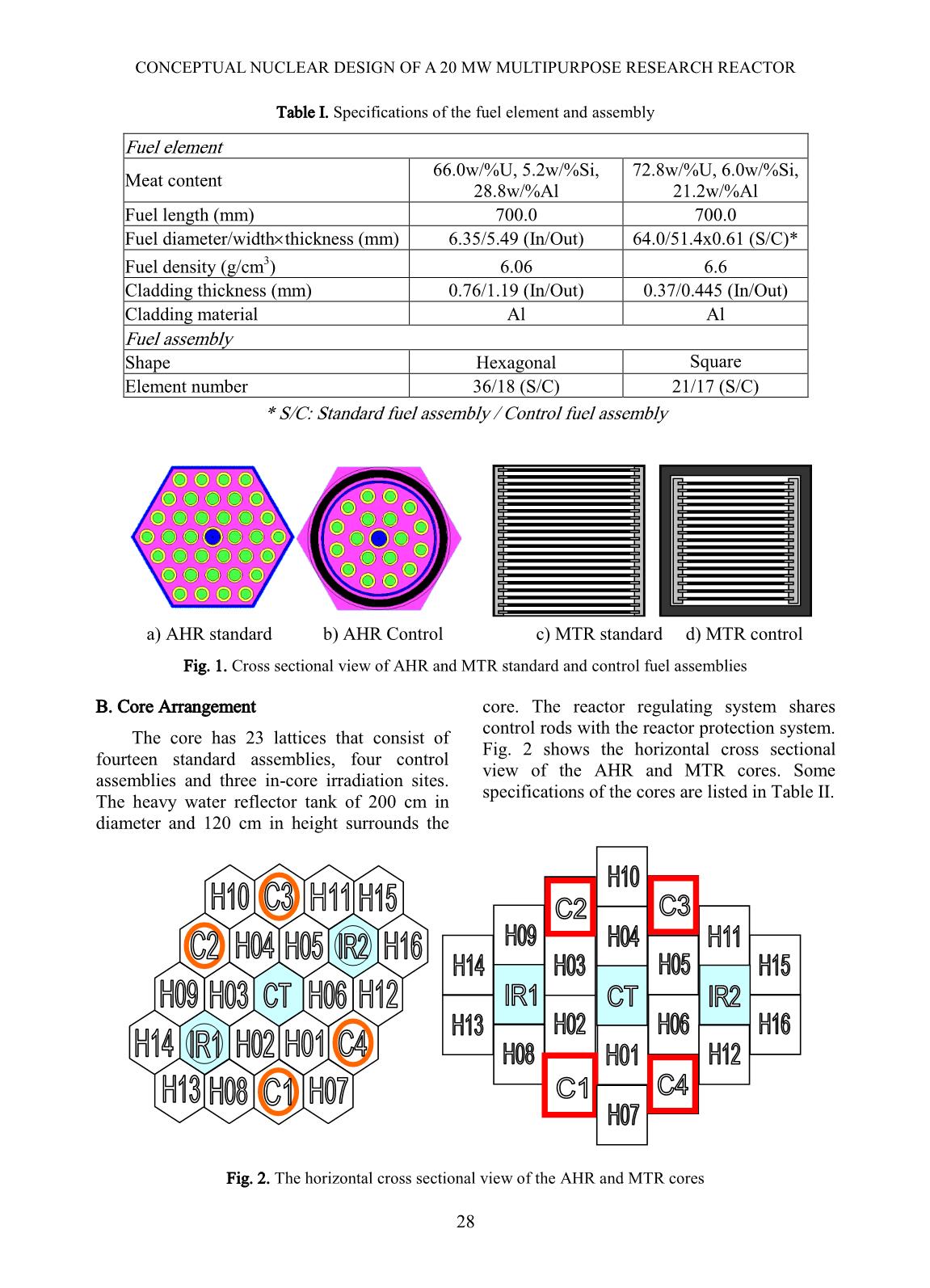
Trang 3
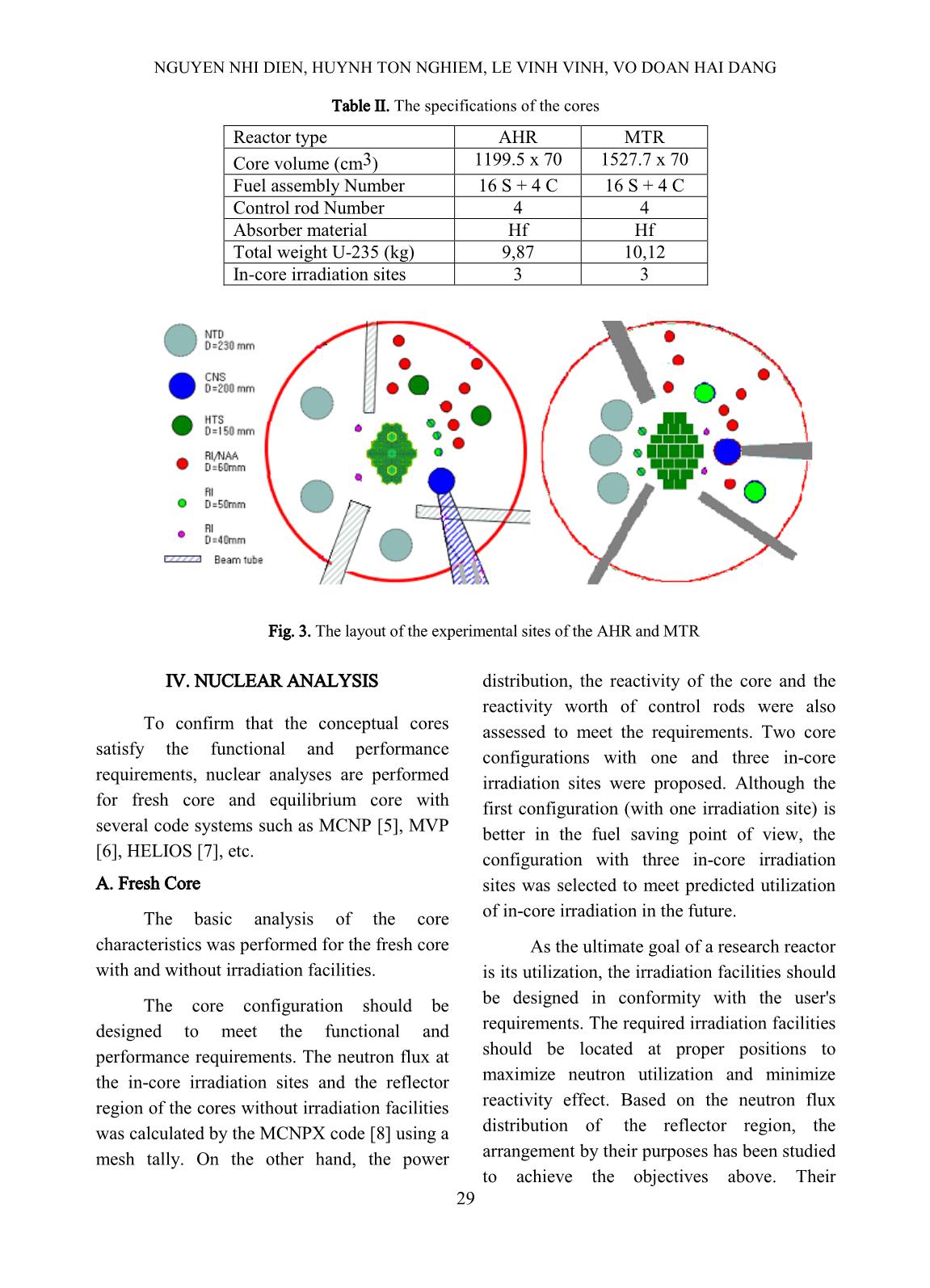
Trang 4
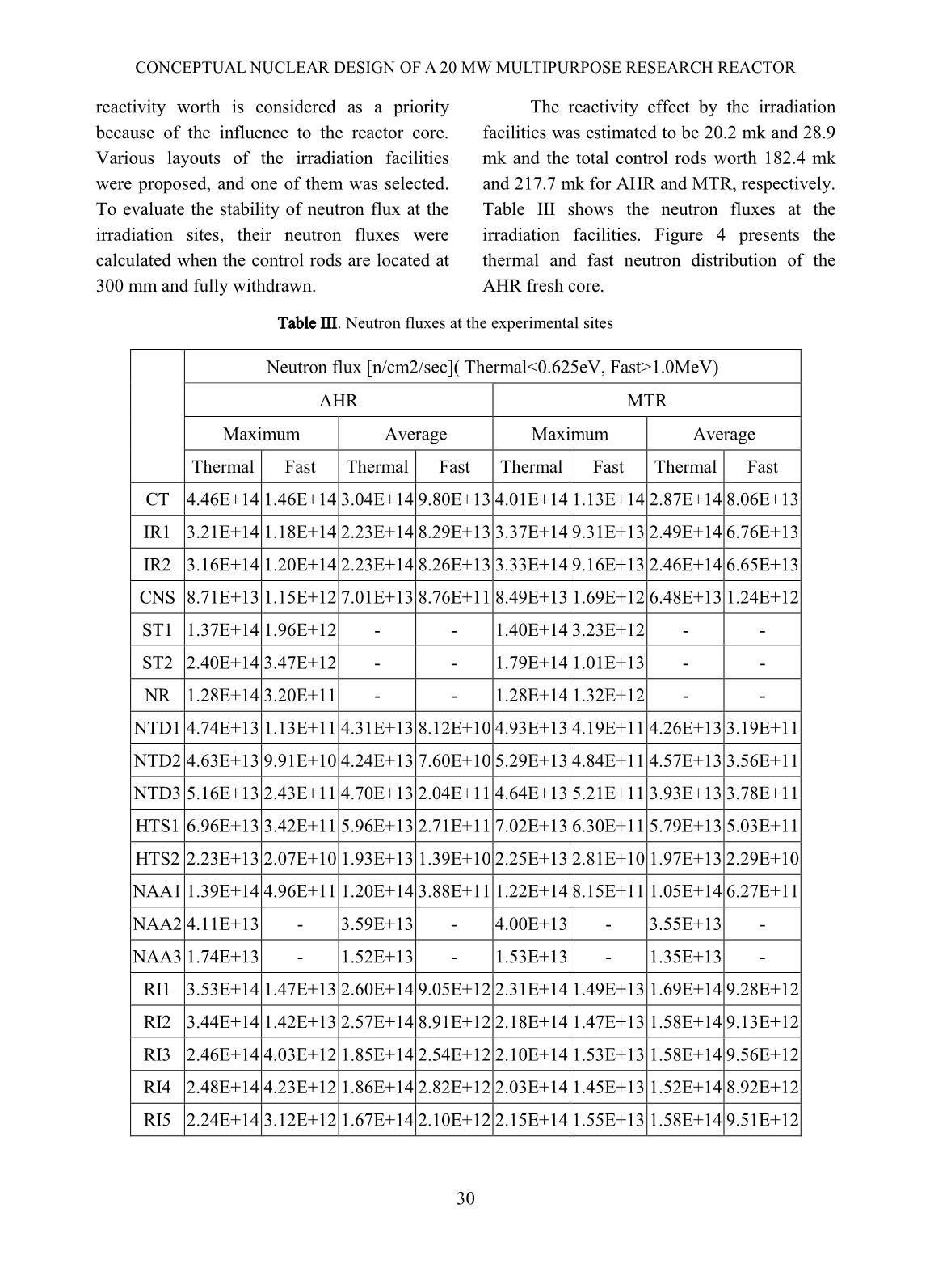
Trang 5
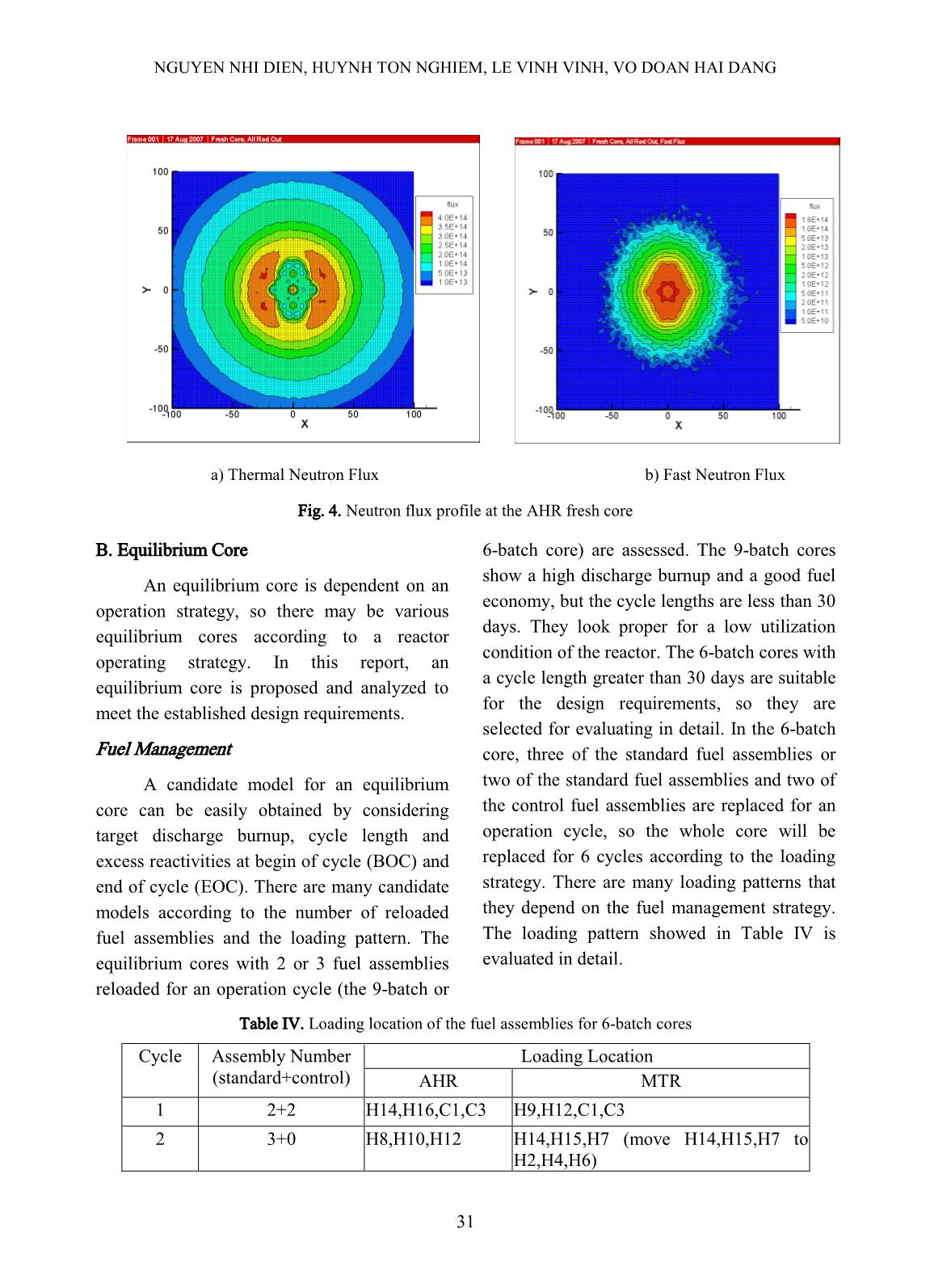
Trang 6
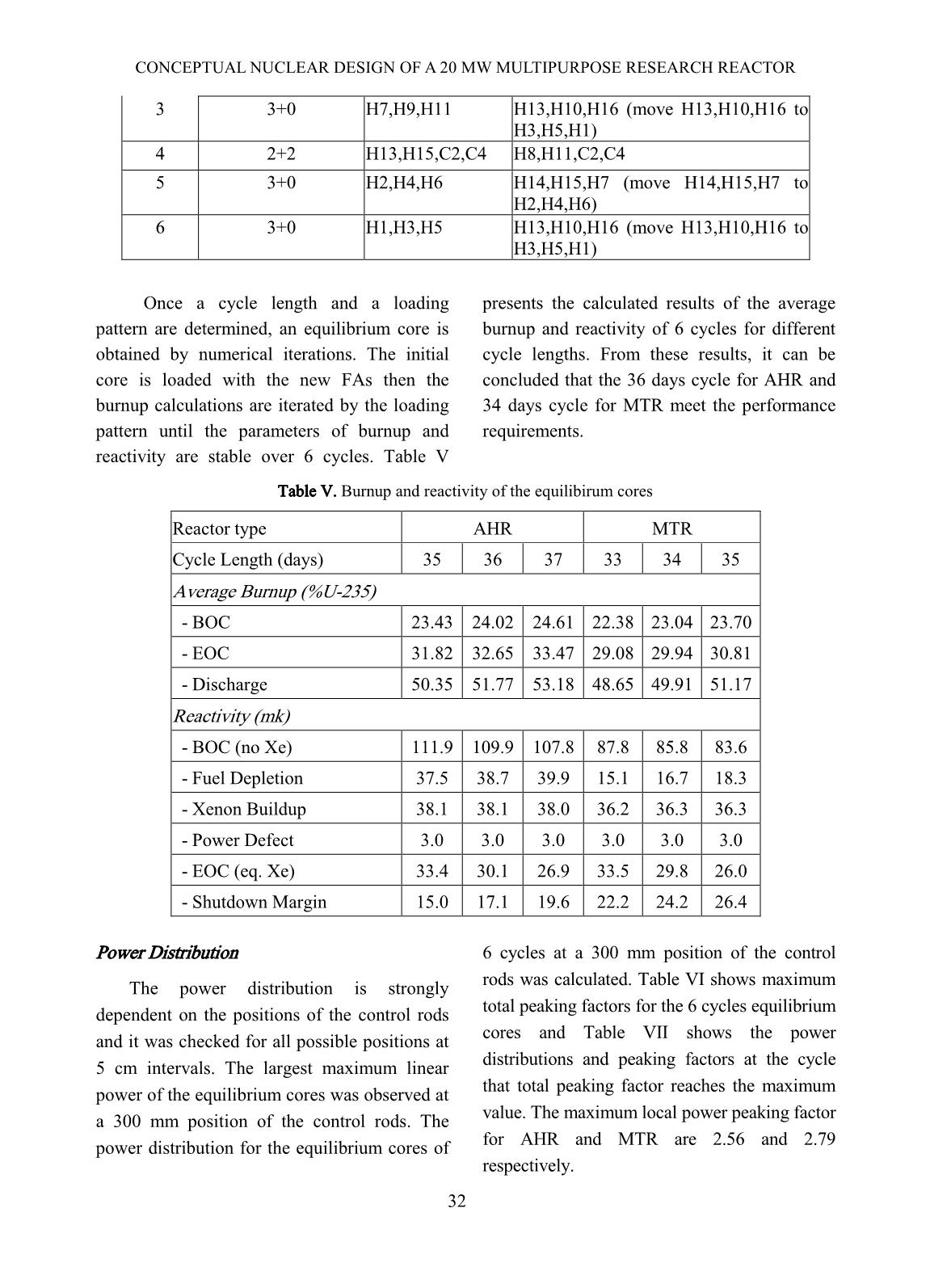
Trang 7
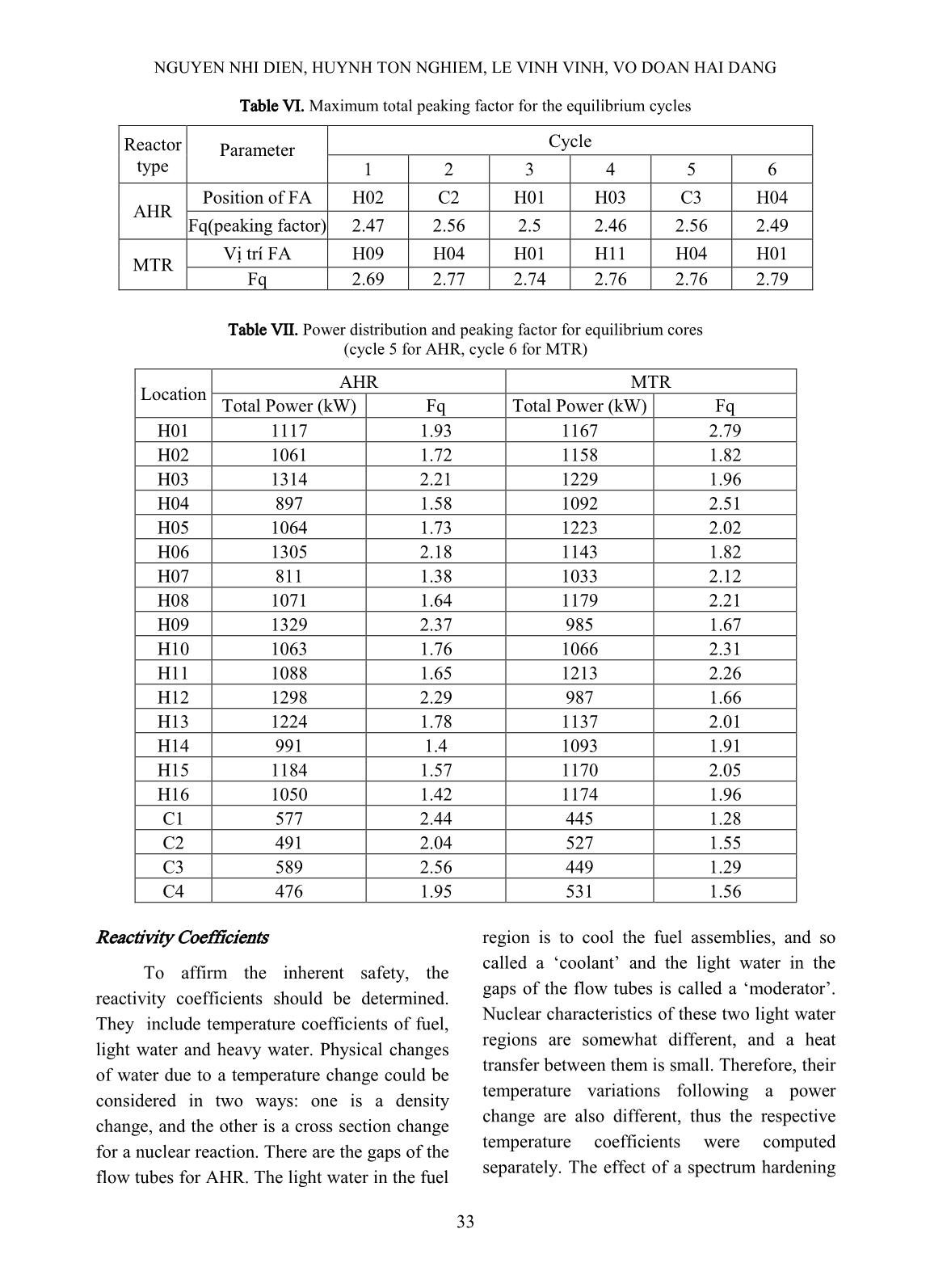
Trang 8
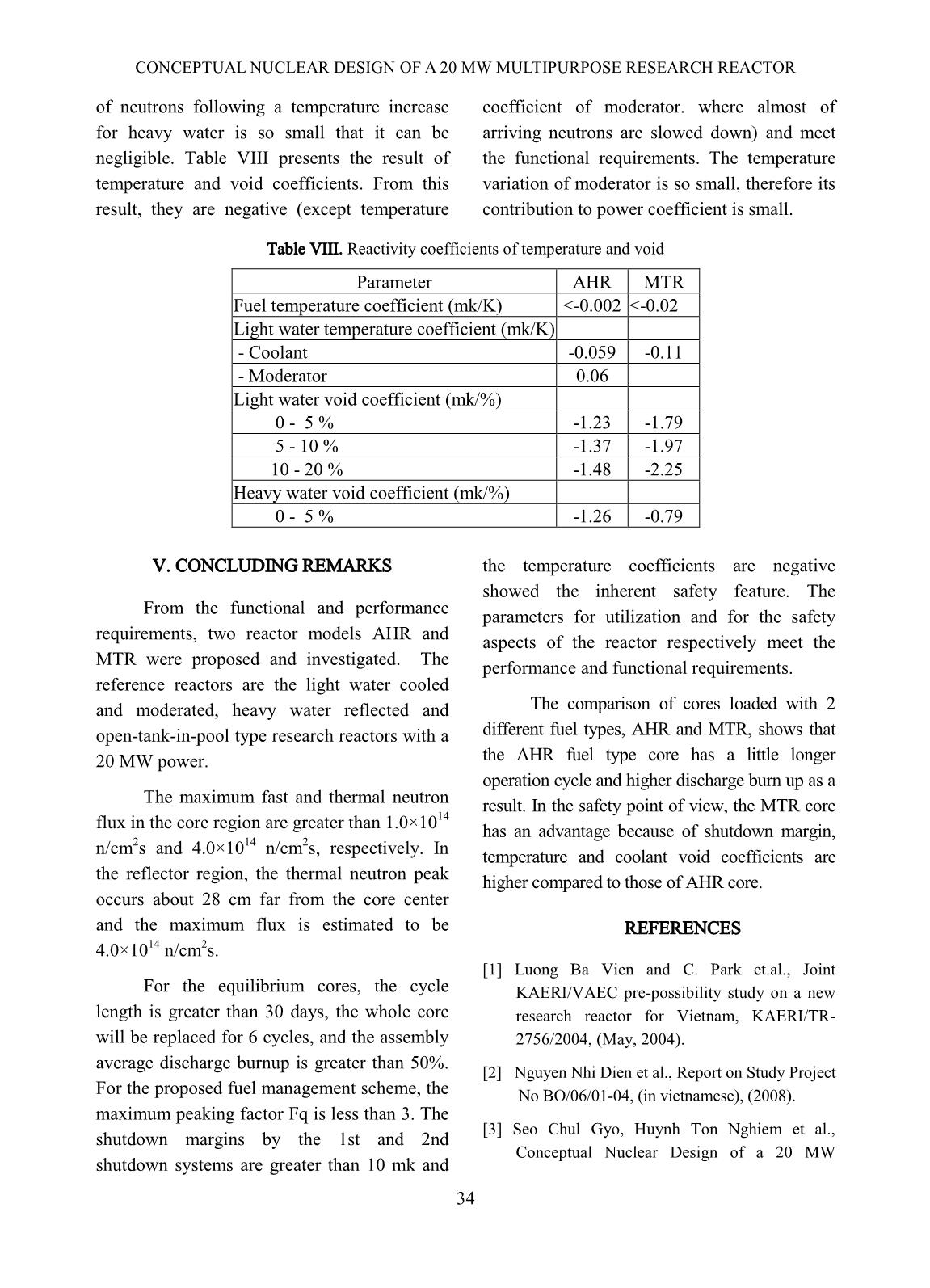
Trang 9
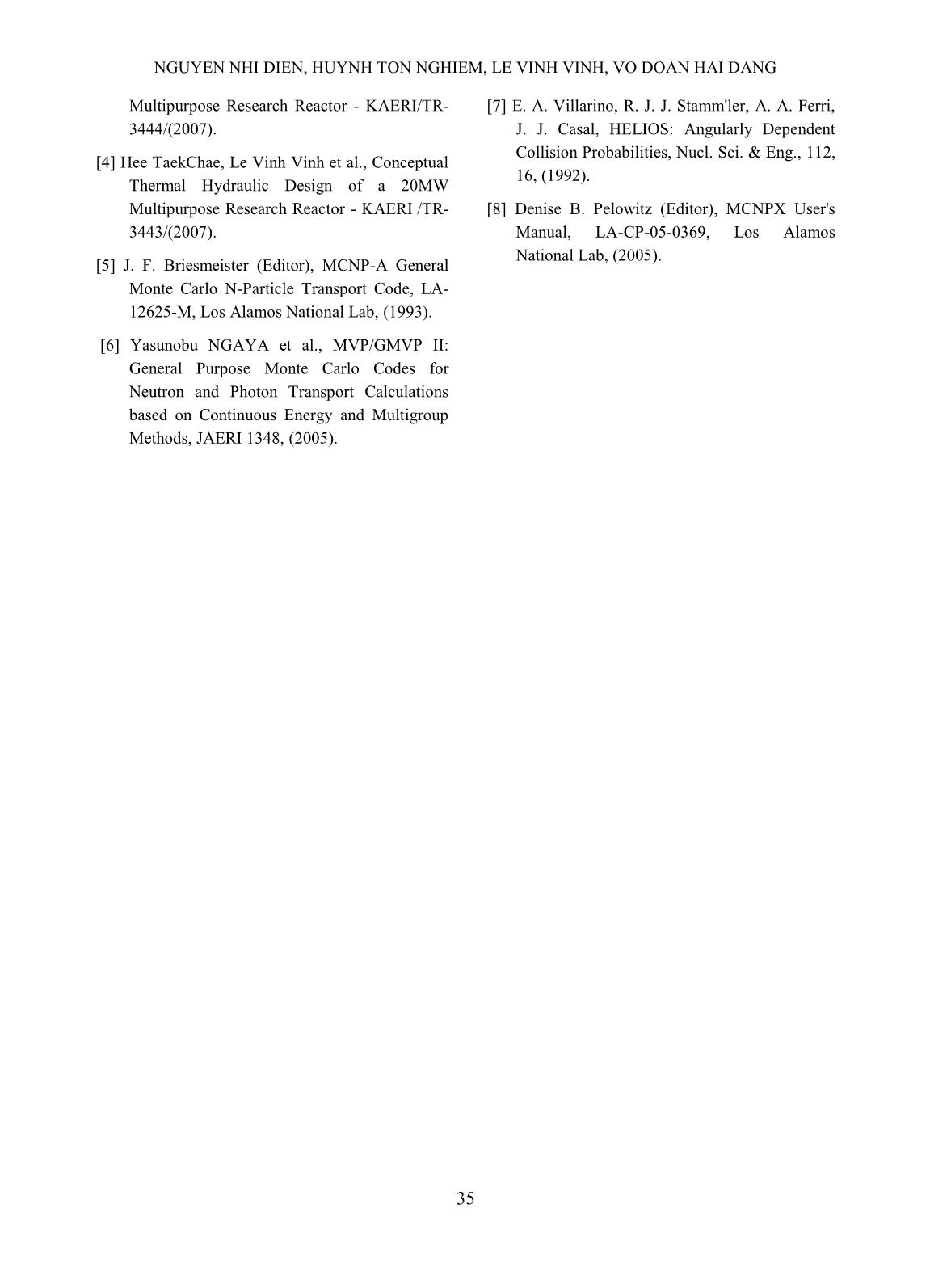
Trang 10
Tóm tắt nội dung tài liệu: Conceptual nuclear design of a 20 MW multipurpose research reactor
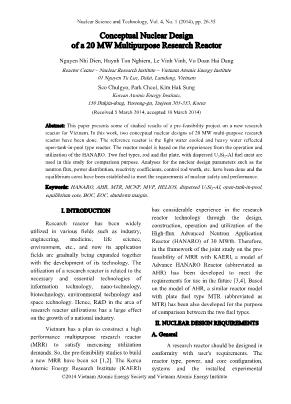
d MTR standard and control fuel assemblies B. Core Arrangement The core has 23 lattices that consist of fourteen standard assemblies, four control assemblies and three in-core irradiation sites. The heavy water reflector tank of 200 cm in diameter and 120 cm in height surrounds the core. The reactor regulating system shares control rods with the reactor protection system. Fig. 2 shows the horizontal cross sectional view of the AHR and MTR cores. Some specifications of the cores are listed in Table II. Fig. 2. The horizontal cross sectional view of the AHR and MTR cores a) AHR standard b) AHR Control c) MTR standard d) MTR control NGUYEN NHI DIEN, HUYNH TON NGHIEM, LE VINH VINH, VO DOAN HAI DANG 29 Table II. The specifications of the cores Reactor type AHR MTR Core volume (cm3) 1199.5 x 70 1527.7 x 70 Fuel assembly Number 16 S + 4 C 16 S + 4 C Control rod Number 4 4 Absorber material Hf Hf Total weight U-235 (kg) 9,87 10,12 In-core irradiation sites 3 3 IV. NUCLEAR ANALYSIS To confirm that the conceptual cores satisfy the functional and performance requirements, nuclear analyses are performed for fresh core and equilibrium core with several code systems such as MCNP [5], MVP [6], HELIOS [7], etc. A. Fresh Core The basic analysis of the core characteristics was performed for the fresh core with and without irradiation facilities. The core configuration should be designed to meet the functional and performance requirements. The neutron flux at the in-core irradiation sites and the reflector region of the cores without irradiation facilities was calculated by the MCNPX code [8] using a mesh tally. On the other hand, the power distribution, the reactivity of the core and the reactivity worth of control rods were also assessed to meet the requirements. Two core configurations with one and three in-core irradiation sites were proposed. Although the first configuration (with one irradiation site) is better in the fuel saving point of view, the configuration with three in-core irradiation sites was selected to meet predicted utilization of in-core irradiation in the future. As the ultimate goal of a research reactor is its utilization, the irradiation facilities should be designed in conformity with the user's requirements. The required irradiation facilities should be located at proper positions to maximize neutron utilization and minimize reactivity effect. Based on the neutron flux distribution of the reflector region, the arrangement by their purposes has been studied to achieve the objectives above. Their Fig. 3. The layout of the experimental sites of the AHR and MTR CONCEPTUAL NUCLEAR DESIGN OF A 20 MW MULTIPURPOSE RESEARCH REACTOR 30 reactivity worth is considered as a priority because of the influence to the reactor core. Various layouts of the irradiation facilities were proposed, and one of them was selected. To evaluate the stability of neutron flux at the irradiation sites, their neutron fluxes were calculated when the control rods are located at 300 mm and fully withdrawn. The reactivity effect by the irradiation facilities was estimated to be 20.2 mk and 28.9 mk and the total control rods worth 182.4 mk and 217.7 mk for AHR and MTR, respectively. Table III shows the neutron fluxes at the irradiation facilities. Figure 4 presents the thermal and fast neutron distribution of the AHR fresh core. Table III. Neutron fluxes at the experimental sites Neutron flux [n/cm2/sec]( Thermal1.0MeV) AHR MTR Maximum Average Maximum Average Thermal Fast Thermal Fast Thermal Fast Thermal Fast CT 4.46E+14 1.46E+14 3.04E+14 9.80E+13 4.01E+14 1.13E+14 2.87E+14 8.06E+13 IR1 3.21E+14 1.18E+14 2.23E+14 8.29E+13 3.37E+14 9.31E+13 2.49E+14 6.76E+13 IR2 3.16E+14 1.20E+14 2.23E+14 8.26E+13 3.33E+14 9.16E+13 2.46E+14 6.65E+13 CNS 8.71E+13 1.15E+12 7.01E+13 8.76E+11 8.49E+13 1.69E+12 6.48E+13 1.24E+12 ST1 1.37E+14 1.96E+12 - - 1.40E+14 3.23E+12 - - ST2 2.40E+14 3.47E+12 - - 1.79E+14 1.01E+13 - - NR 1.28E+14 3.20E+11 - - 1.28E+14 1.32E+12 - - NTD1 4.74E+13 1.13E+11 4.31E+13 8.12E+10 4.93E+13 4.19E+11 4.26E+13 3.19E+11 NTD2 4.63E+13 9.91E+10 4.24E+13 7.60E+10 5.29E+13 4.84E+11 4.57E+13 3.56E+11 NTD3 5.16E+13 2.43E+11 4.70E+13 2.04E+11 4.64E+13 5.21E+11 3.93E+13 3.78E+11 HTS1 6.96E+13 3.42E+11 5.96E+13 2.71E+11 7.02E+13 6.30E+11 5.79E+13 5.03E+11 HTS2 2.23E+13 2.07E+10 1.93E+13 1.39E+10 2.25E+13 2.81E+10 1.97E+13 2.29E+10 NAA1 1.39E+14 4.96E+11 1.20E+14 3.88E+11 1.22E+14 8.15E+11 1.05E+14 6.27E+11 NAA2 4.11E+13 - 3.59E+13 - 4.00E+13 - 3.55E+13 - NAA3 1.74E+13 - 1.52E+13 - 1.53E+13 - 1.35E+13 - RI1 3.53E+14 1.47E+13 2.60E+14 9.05E+12 2.31E+14 1.49E+13 1.69E+14 9.28E+12 RI2 3.44E+14 1.42E+13 2.57E+14 8.91E+12 2.18E+14 1.47E+13 1.58E+14 9.13E+12 RI3 2.46E+14 4.03E+12 1.85E+14 2.54E+12 2.10E+14 1.53E+13 1.58E+14 9.56E+12 RI4 2.48E+14 4.23E+12 1.86E+14 2.82E+12 2.03E+14 1.45E+13 1.52E+14 8.92E+12 RI5 2.24E+14 3.12E+12 1.67E+14 2.10E+12 2.15E+14 1.55E+13 1.58E+14 9.51E+12 NGUYEN NHI DIEN, HUYNH TON NGHIEM, LE VINH VINH, VO DOAN HAI DANG 31 Fig. 4. Neutron flux profile at the AHR fresh core B. Equilibrium Core An equilibrium core is dependent on an operation strategy, so there may be various equilibrium cores according to a reactor operating strategy. In this report, an equilibrium core is proposed and analyzed to meet the established design requirements. Fuel Management A candidate model for an equilibrium core can be easily obtained by considering target discharge burnup, cycle length and excess reactivities at begin of cycle (BOC) and end of cycle (EOC). There are many candidate models according to the number of reloaded fuel assemblies and the loading pattern. The equilibrium cores with 2 or 3 fuel assemblies reloaded for an operation cycle (the 9-batch or 6-batch core) are assessed. The 9-batch cores show a high discharge burnup and a good fuel economy, but the cycle lengths are less than 30 days. They look proper for a low utilization condition of the reactor. The 6-batch cores with a cycle length greater than 30 days are suitable for the design requirements, so they are selected for evaluating in detail. In the 6-batch core, three of the standard fuel assemblies or two of the standard fuel assemblies and two of the control fuel assemblies are replaced for an operation cycle, so the whole core will be replaced for 6 cycles according to the loading strategy. There are many loading patterns that they depend on the fuel management strategy. The loading pattern showed in Table IV is evaluated in detail. Table IV. Loading location of the fuel assemblies for 6-batch cores Cycle Assembly Number (standard+control) Loading Location AHR MTR 1 2+2 H14,H16,C1,C3 H9,H12,C1,C3 2 3+0 H8,H10,H12 H14,H15,H7 (move H14,H15,H7 to H2,H4,H6) a) Thermal Neutron Flux b) Fast Neutron Flux CONCEPTUAL NUCLEAR DESIGN OF A 20 MW MULTIPURPOSE RESEARCH REACTOR 30 3 3+0 H7,H9,H11 H13,H10,H16 (move H13,H10,H16 to H3,H5,H1) 4 2+2 H13,H15,C2,C4 H8,H11,C2,C4 5 3+0 H2,H4,H6 H14,H15,H7 (move H14,H15,H7 to H2,H4,H6) 6 3+0 H1,H3,H5 H13,H10,H16 (move H13,H10,H16 to H3,H5,H1) Once a cycle length and a loading pattern are determined, an equilibrium core is obtained by numerical iterations. The initial core is loaded with the new FAs then the burnup calculations are iterated by the loading pattern until the parameters of burnup and reactivity are stable over 6 cycles. Table V presents the calculated results of the average burnup and reactivity of 6 cycles for different cycle lengths. From these results, it can be concluded that the 36 days cycle for AHR and 34 days cycle for MTR meet the performance requirements. Table V. Burnup and reactivity of the equilibirum cores Reactor type AHR MTR Cycle Length (days) 35 36 37 33 34 35 Average Burnup (%U-235) - BOC 23.43 24.02 24.61 22.38 23.04 23.70 - EOC 31.82 32.65 33.47 29.08 29.94 30.81 - Discharge 50.35 51.77 53.18 48.65 49.91 51.17 Reactivity (mk) - BOC (no Xe) 111.9 109.9 107.8 87.8 85.8 83.6 - Fuel Depletion 37.5 38.7 39.9 15.1 16.7 18.3 - Xenon Buildup 38.1 38.1 38.0 36.2 36.3 36.3 - Power Defect 3.0 3.0 3.0 3.0 3.0 3.0 - EOC (eq. Xe) 33.4 30.1 26.9 33.5 29.8 26.0 - Shutdown Margin 15.0 17.1 19.6 22.2 24.2 26.4 Power Distribution The power distribution is strongly dependent on the positions of the control rods and it was checked for all possible positions at 5 cm intervals. The largest maximum linear power of the equilibrium cores was observed at a 300 mm position of the control rods. The power distribution for the equilibrium cores of 6 cycles at a 300 mm position of the control rods was calculated. Table VI shows maximum total peaking factors for the 6 cycles equilibrium cores and Table VII shows the power distributions and peaking factors at the cycle that total peaking factor reaches the maximum value. The maximum local power peaking factor for AHR and MTR are 2.56 and 2.79 respectively. 32 NGUYEN NHI DIEN, HUYNH TON NGHIEM, LE VINH VINH, VO DOAN HAI DANG 33 Table VI. Maximum total peaking factor for the equilibrium cycles Reactor type Parameter Cycle 1 2 3 4 5 6 AHR Position of FA H02 C2 H01 H03 C3 H04 Fq(peaking factor) 2.47 2.56 2.5 2.46 2.56 2.49 MTR Vị trí FA H09 H04 H01 H11 H04 H01 Fq 2.69 2.77 2.74 2.76 2.76 2.79 Table VII. Power distribution and peaking factor for equilibrium cores (cycle 5 for AHR, cycle 6 for MTR) Location AHR MTR Total Power (kW) Fq Total Power (kW) Fq H01 1117 1.93 1167 2.79 H02 1061 1.72 1158 1.82 H03 1314 2.21 1229 1.96 H04 897 1.58 1092 2.51 H05 1064 1.73 1223 2.02 H06 1305 2.18 1143 1.82 H07 811 1.38 1033 2.12 H08 1071 1.64 1179 2.21 H09 1329 2.37 985 1.67 H10 1063 1.76 1066 2.31 H11 1088 1.65 1213 2.26 H12 1298 2.29 987 1.66 H13 1224 1.78 1137 2.01 H14 991 1.4 1093 1.91 H15 1184 1.57 1170 2.05 H16 1050 1.42 1174 1.96 C1 577 2.44 445 1.28 C2 491 2.04 527 1.55 C3 589 2.56 449 1.29 C4 476 1.95 531 1.56 Reactivity Coefficients To affirm the inherent safety, the reactivity coefficients should be determined. They include temperature coefficients of fuel, light water and heavy water. Physical changes of water due to a temperature change could be considered in two ways: one is a density change, and the other is a cross section change for a nuclear reaction. There are the gaps of the flow tubes for AHR. The light water in the fuel region is to cool the fuel assemblies, and so called a ‘coolant’ and the light water in the gaps of the flow tubes is called a ‘moderator’. Nuclear characteristics of these two light water regions are somewhat different, and a heat transfer between them is small. Therefore, their temperature variations following a power change are also different, thus the respective temperature coefficients were computed separately. The effect of a spectrum hardening CONCEPTUAL NUCLEAR DESIGN OF A 20 MW MULTIPURPOSE RESEARCH REACTOR 34 of neutrons following a temperature increase for heavy water is so small that it can be negligible. Table VIII presents the result of temperature and void coefficients. From this result, they are negative (except temperature coefficient of moderator. where almost of arriving neutrons are slowed down) and meet the functional requirements. The temperature variation of moderator is so small, therefore its contribution to power coefficient is small. Table VIII. Reactivity coefficients of temperature and void Parameter AHR MTR Fuel temperature coefficient (mk/K) <-0.002 <-0.02 Light water temperature coefficient (mk/K) - Coolant -0.059 -0.11 - Moderator 0.06 Light water void coefficient (mk/%) 0 - 5 % -1.23 -1.79 5 - 10 % -1.37 -1.97 10 - 20 % -1.48 -2.25 Heavy water void coefficient (mk/%) 0 - 5 % -1.26 -0.79 V. CONCLUDING REMARKS From the functional and performance requirements, two reactor models AHR and MTR were proposed and investigated. The reference reactors are the light water cooled and moderated, heavy water reflected and open-tank-in-pool type research reactors with a 20 MW power. The maximum fast and thermal neutron flux in the core region are greater than 1.0×10 14 n/cm 2 s and 4.0×10 14 n/cm 2 s, respectively. In the reflector region, the thermal neutron peak occurs about 28 cm far from the core center and the maximum flux is estimated to be 4.0×10 14 n/cm 2 s. For the equilibrium cores, the cycle length is greater than 30 days, the whole core will be replaced for 6 cycles, and the assembly average discharge burnup is greater than 50%. For the proposed fuel management scheme, the maximum peaking factor Fq is less than 3. The shutdown margins by the 1st and 2nd shutdown systems are greater than 10 mk and the temperature coefficients are negative showed the inherent safety feature. The parameters for utilization and for the safety aspects of the reactor respectively meet the performance and functional requirements. The comparison of cores loaded with 2 different fuel types, AHR and MTR, shows that the AHR fuel type core has a little longer operation cycle and higher discharge burn up as a result. In the safety point of view, the MTR core has an advantage because of shutdown margin, temperature and coolant void coefficients are higher compared to those of AHR core. REFERENCES [1] Luong Ba Vien and C. Park et.al., Joint KAERI/VAEC pre-possibility study on a new research reactor for Vietnam, KAERI/TR- 2756/2004, (May, 2004). [2] Nguyen Nhi Dien et al., Report on Study Project No BO/06/01-04, (in vietnamese), (2008). [3] Seo Chul Gyo, Huynh Ton Nghiem et al., Conceptual Nuclear Design of a 20 MW NGUYEN NHI DIEN, HUYNH TON NGHIEM, LE VINH VINH, VO DOAN HAI DANG 35 Multipurpose Research Reactor - KAERI/TR- 3444/(2007). [4] Hee TaekChae, Le Vinh Vinh et al., Conceptual Thermal Hydraulic Design of a 20MW Multipurpose Research Reactor - KAERI /TR- 3443/(2007). [5] J. F. Briesmeister (Editor), MCNP-A General Monte Carlo N-Particle Transport Code, LA- 12625-M, Los Alamos National Lab, (1993). [6] Yasunobu NGAYA et al., MVP/GMVP II: General Purpose Monte Carlo Codes for Neutron and Photon Transport Calculations based on Continuous Energy and Multigroup Methods, JAERI 1348, (2005). [7] E. A. Villarino, R. J. J. Stamm'ler, A. A. Ferri, J. J. Casal, HELIOS: Angularly Dependent Collision Probabilities, Nucl. Sci. & Eng., 112, 16, (1992). [8] Denise B. Pelowitz (Editor), MCNPX User's Manual, LA-CP-05-0369, Los Alamos National Lab, (2005).
File đính kèm:
conceptual_nuclear_design_of_a_20_mw_multipurpose_research_r.pdf