Calculation results for enhancing ability of I-131 radioisotope production using tellurium dioxide target on the dalat nuclear research reactor
The paper presents the calculation results in re-design of neutron trap of the Dalat Nuclear
Research Reactor (DNRR) for I-131 radioisotope production using TeO2 target. The new design
permits for loading more TeO2 capsules from 9 to 12, 15 and 18 in the neutron trap. The enhancement
of radioisotope production was implemented by re-arrangement of the neutron trap without changing
the dimension or geometry of irradiation capsules. By using neutronics computer code as MCNP6, the
obtained calculation results of I-131 activity in 6 investigated cases showed that the new design by the
re-arrangement of the neutron trap can be used effectively for radioisotope production with thermal
neutron flux in average range from 5.3×1012 to 1×1013 n/cm2.s and the total activity of I-131 isotope
was increased from about 19.2% to 38.8% comparing with the original design using 9 capsules. The
negative reactivity insertion was from 0.60 βeff to 0.96 βeff when loading capsules that also met the
safety requirements of operational conditions of the DNRR.
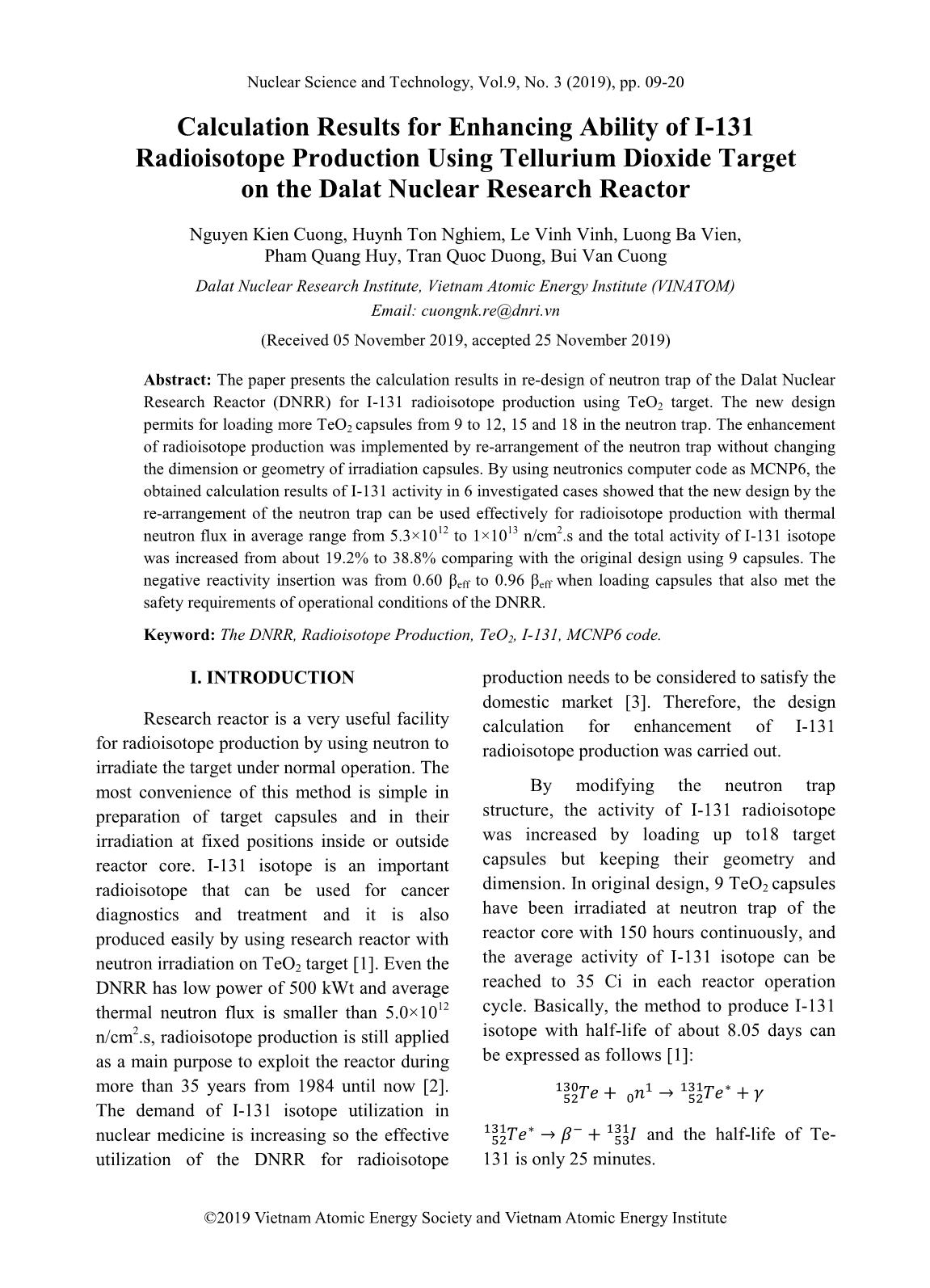
Trang 1
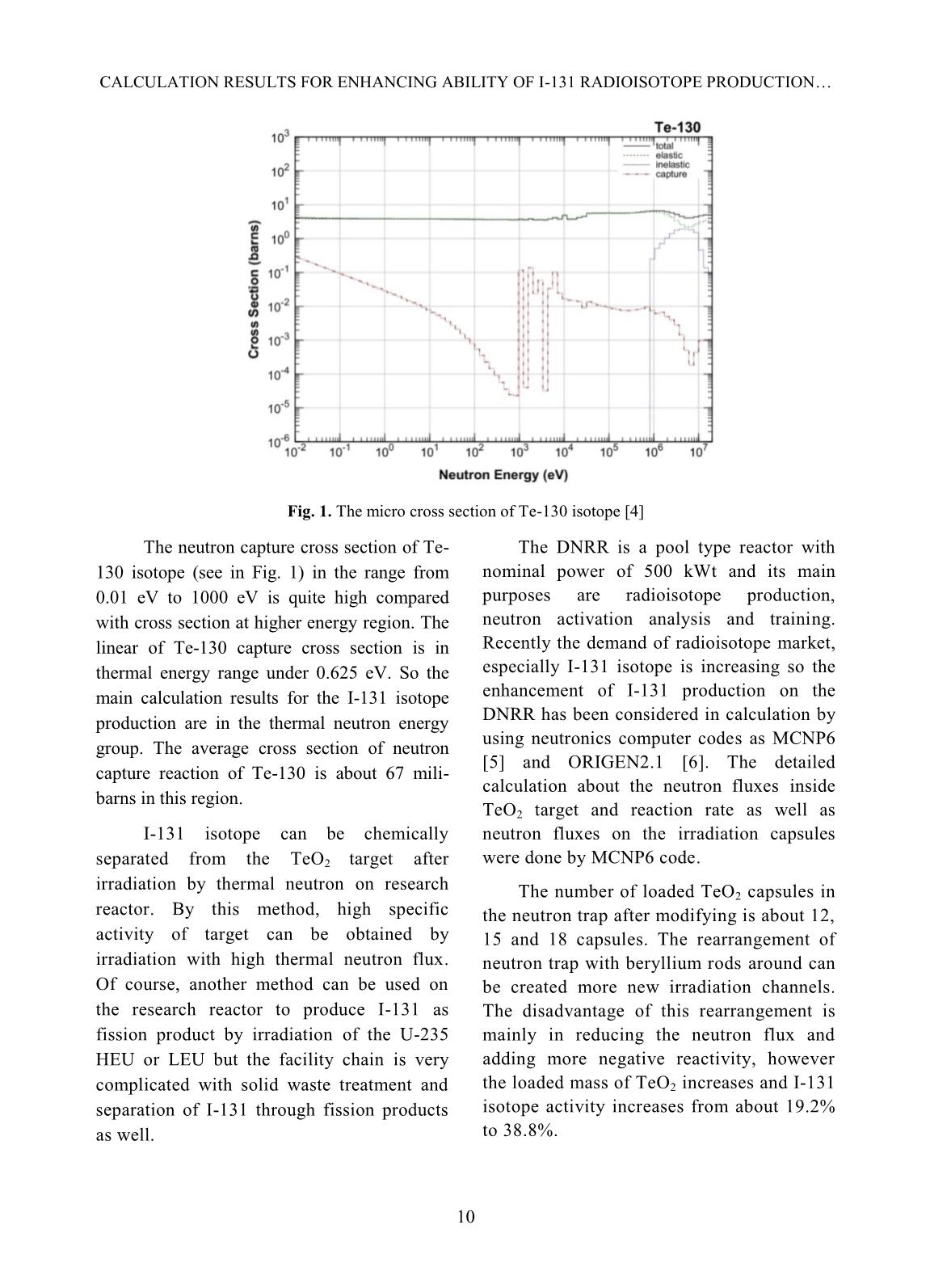
Trang 2
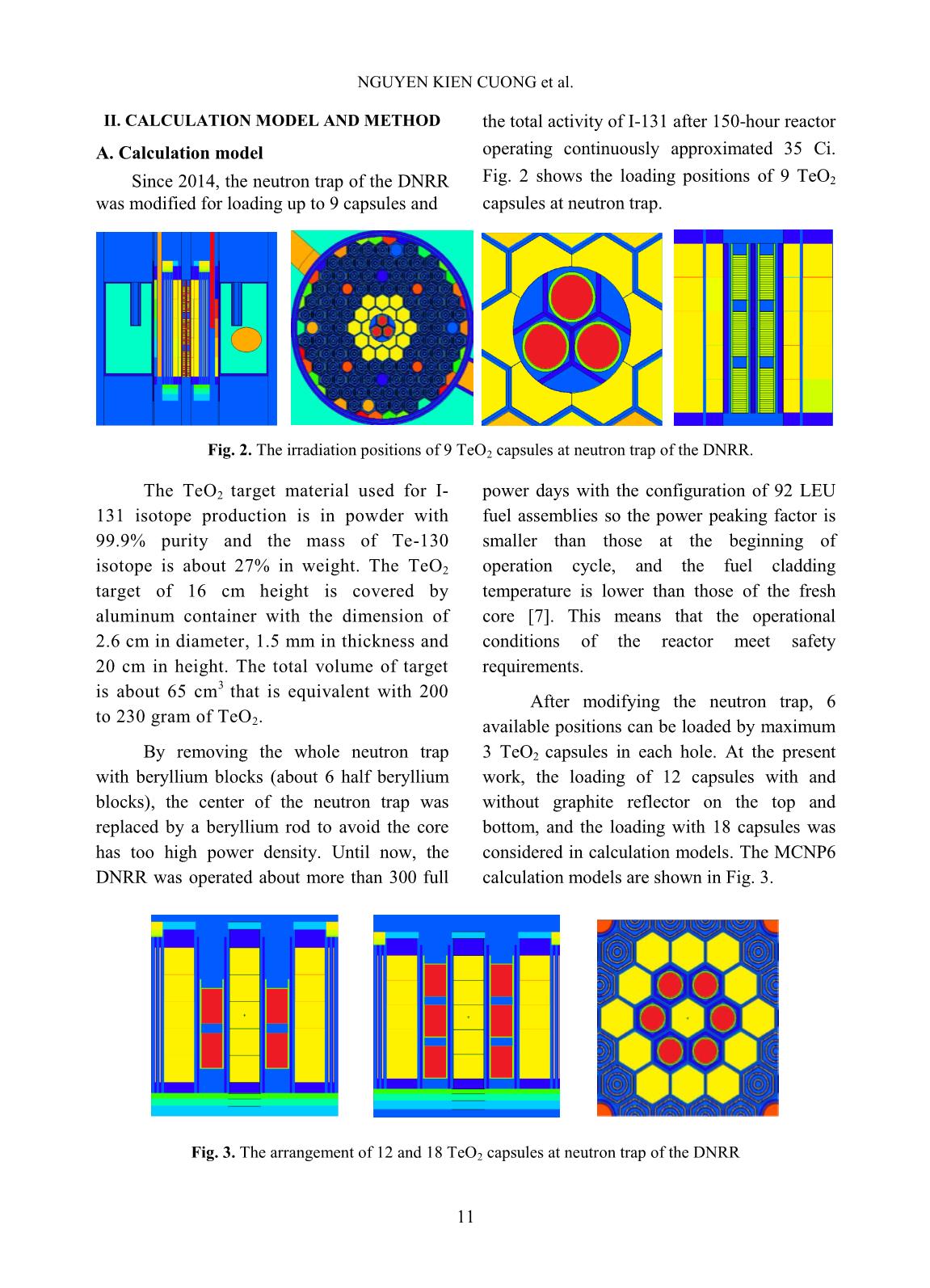
Trang 3
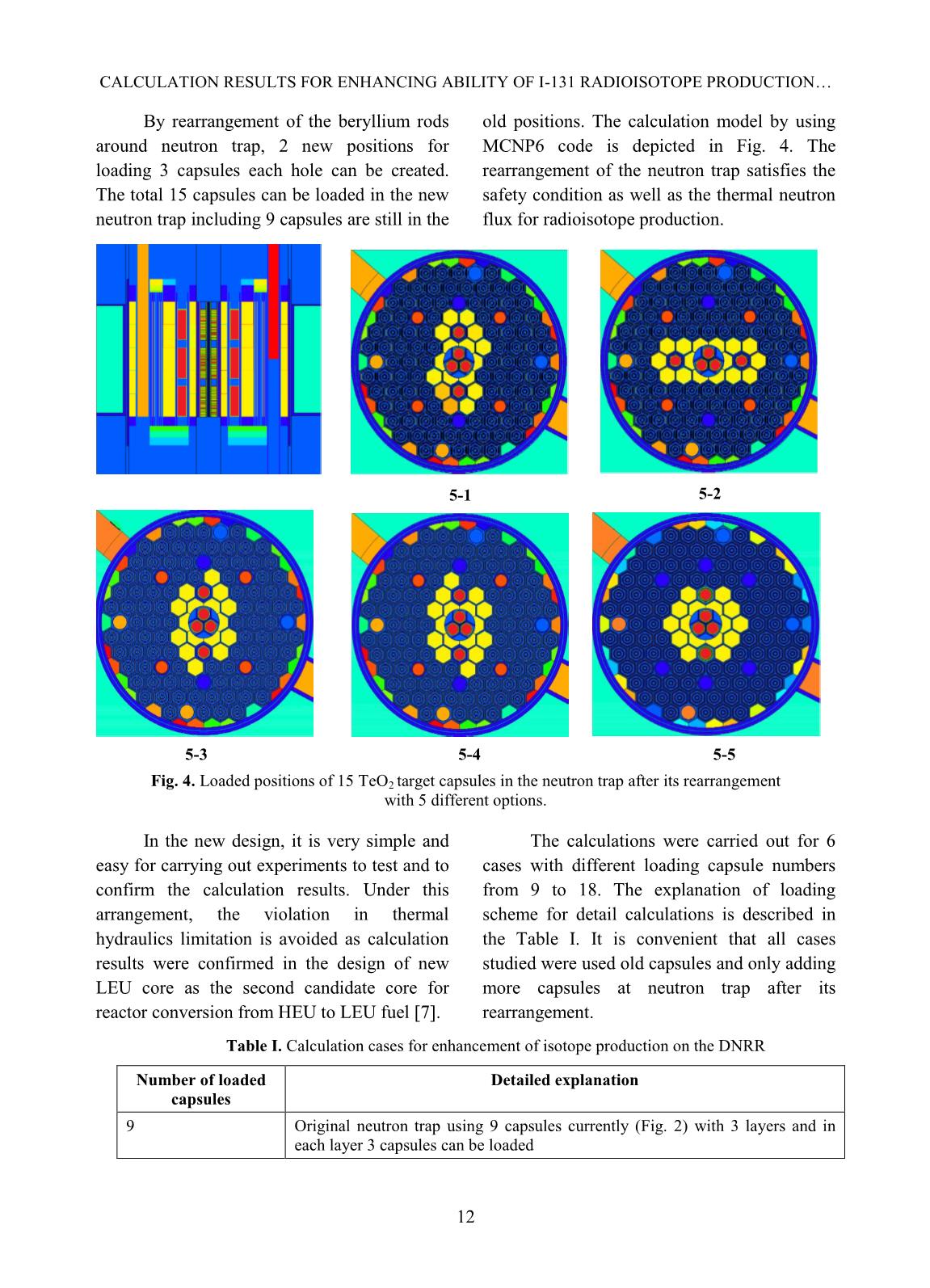
Trang 4
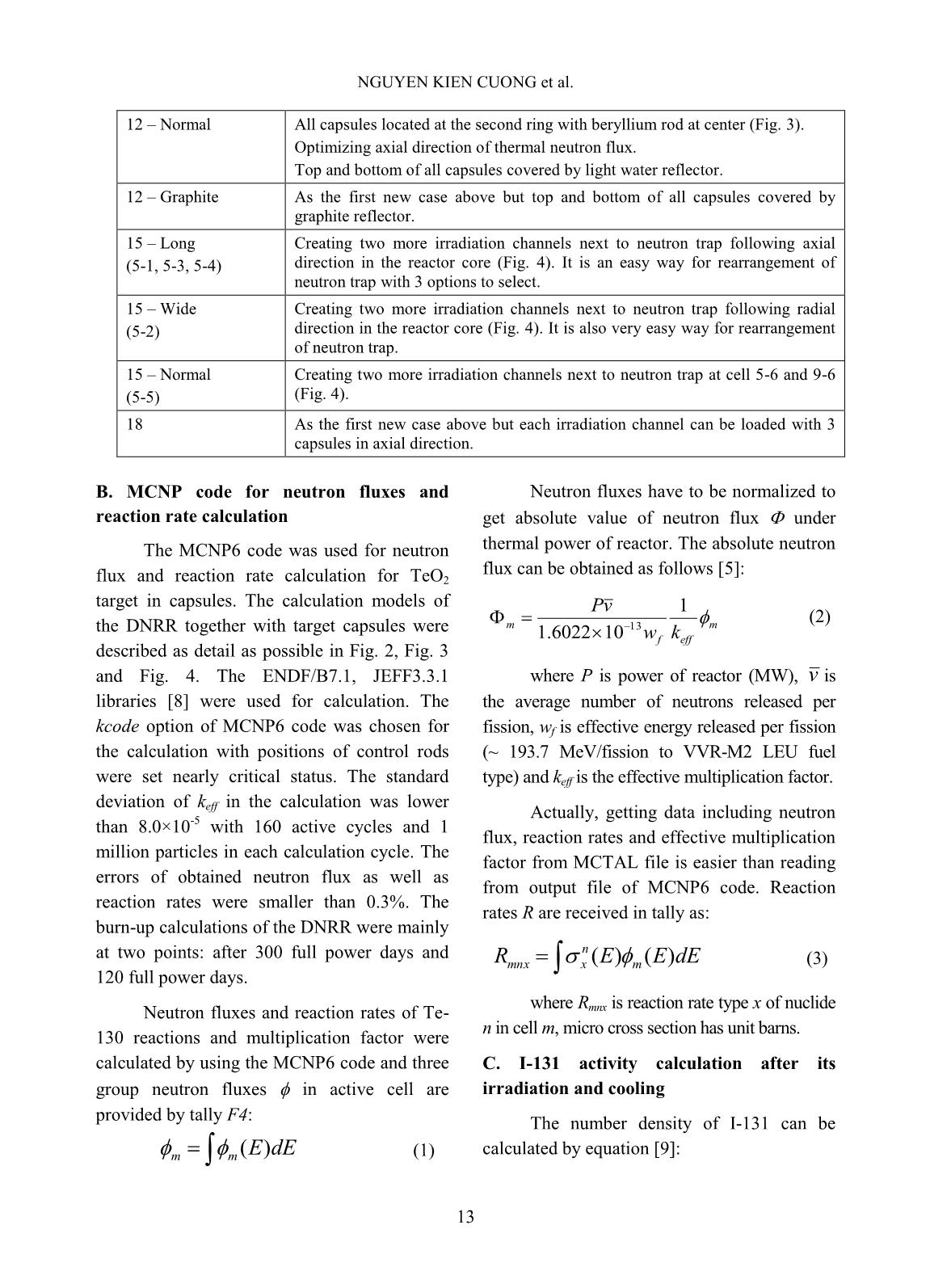
Trang 5
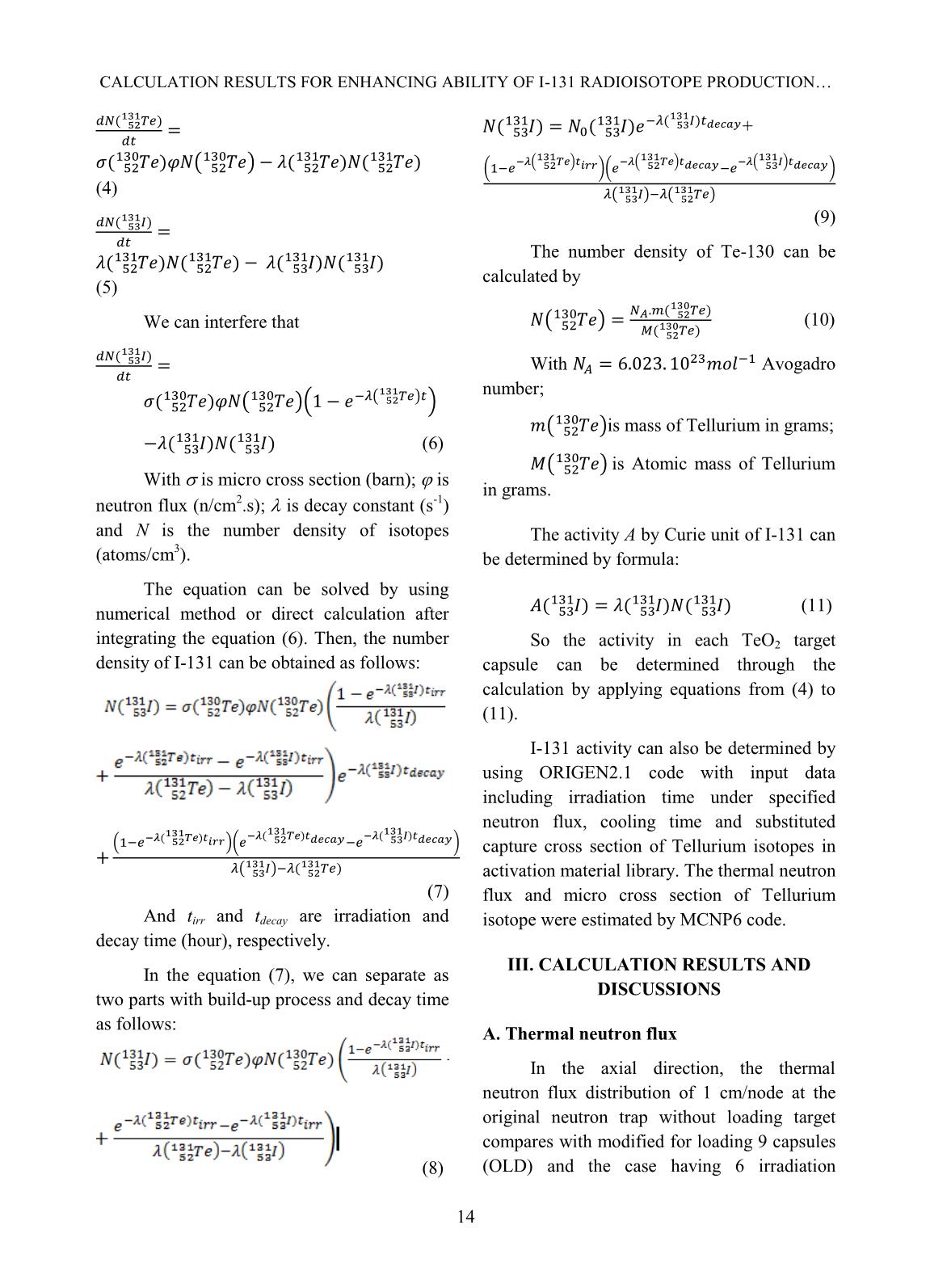
Trang 6
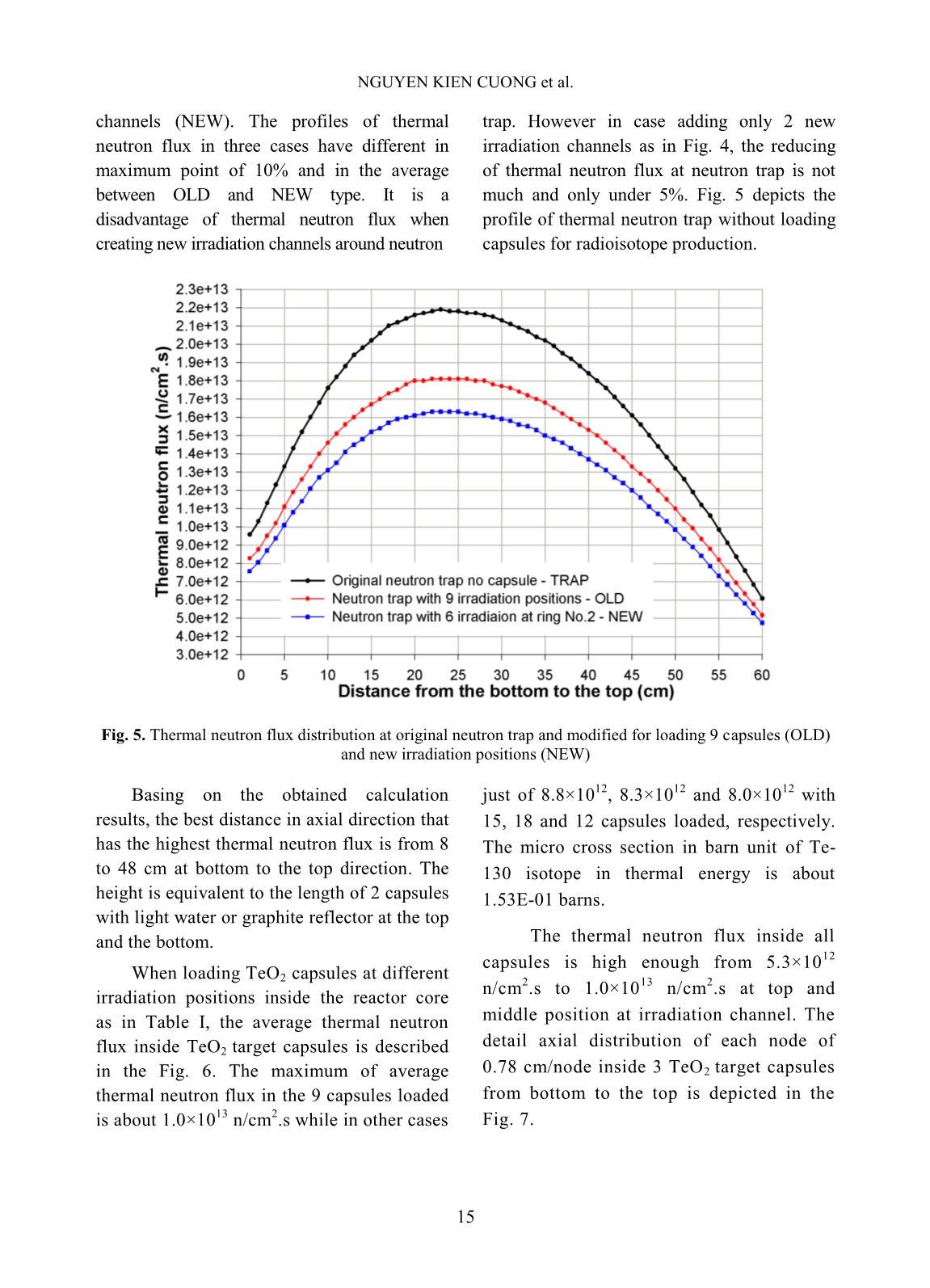
Trang 7
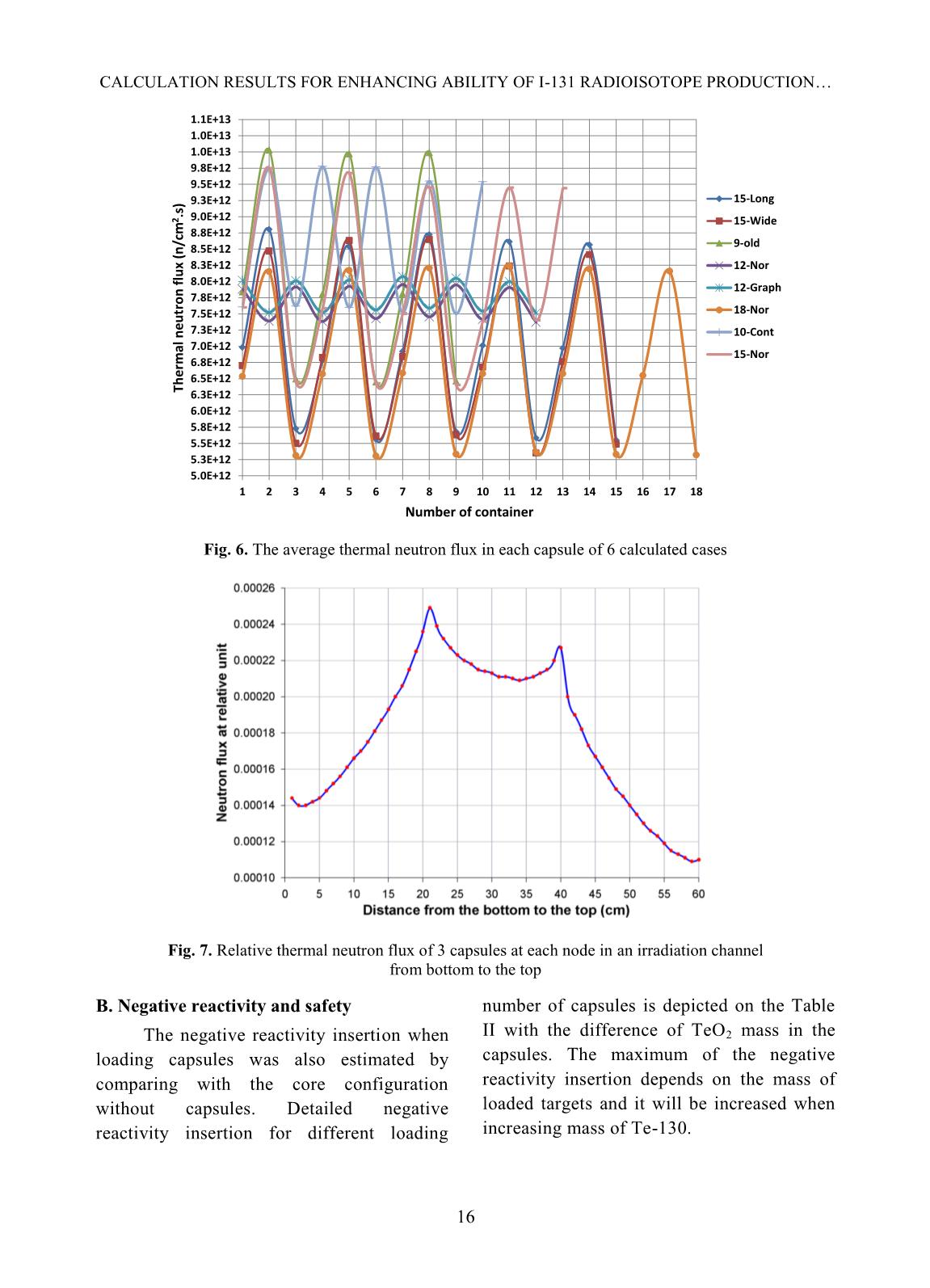
Trang 8
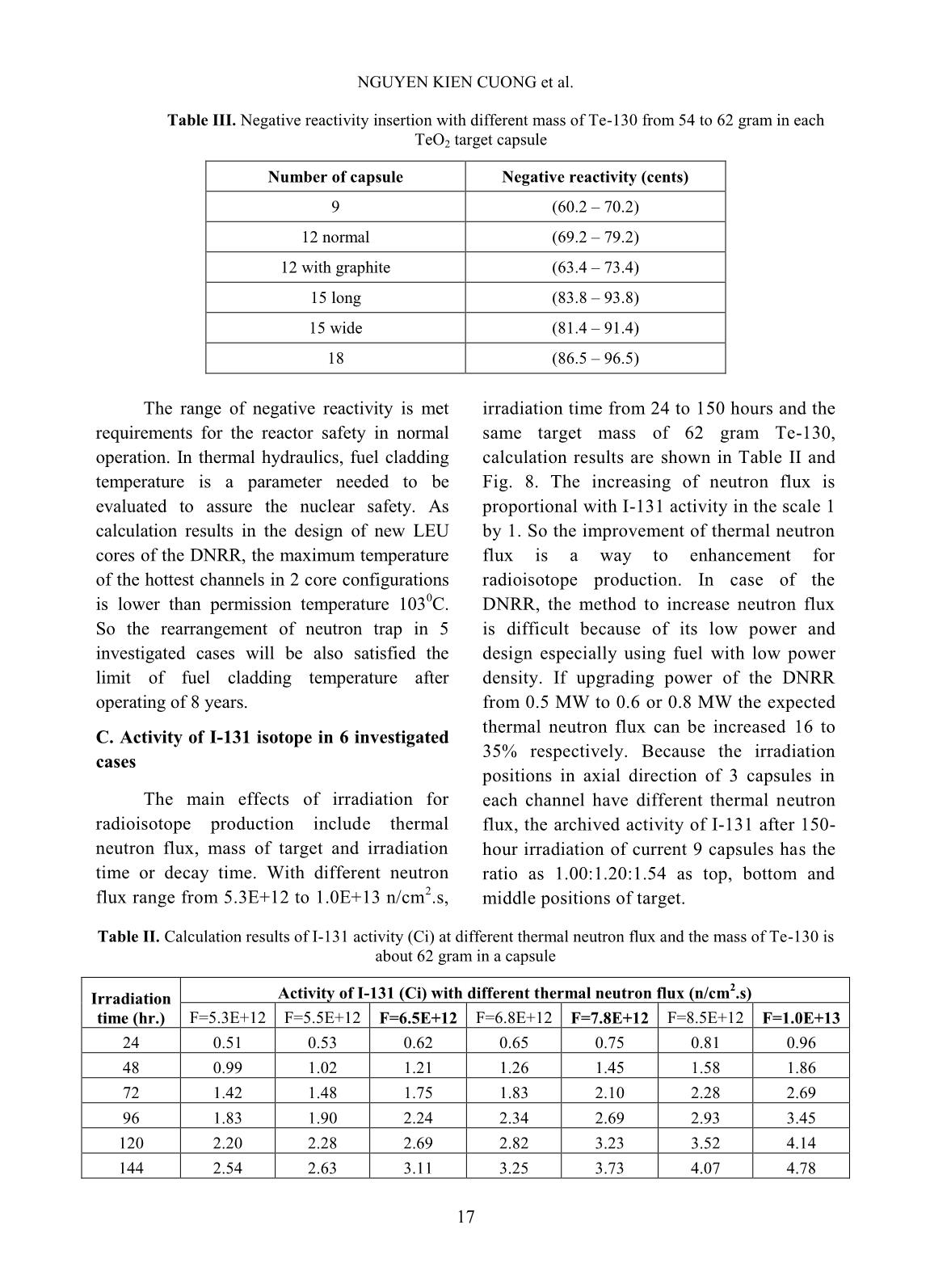
Trang 9
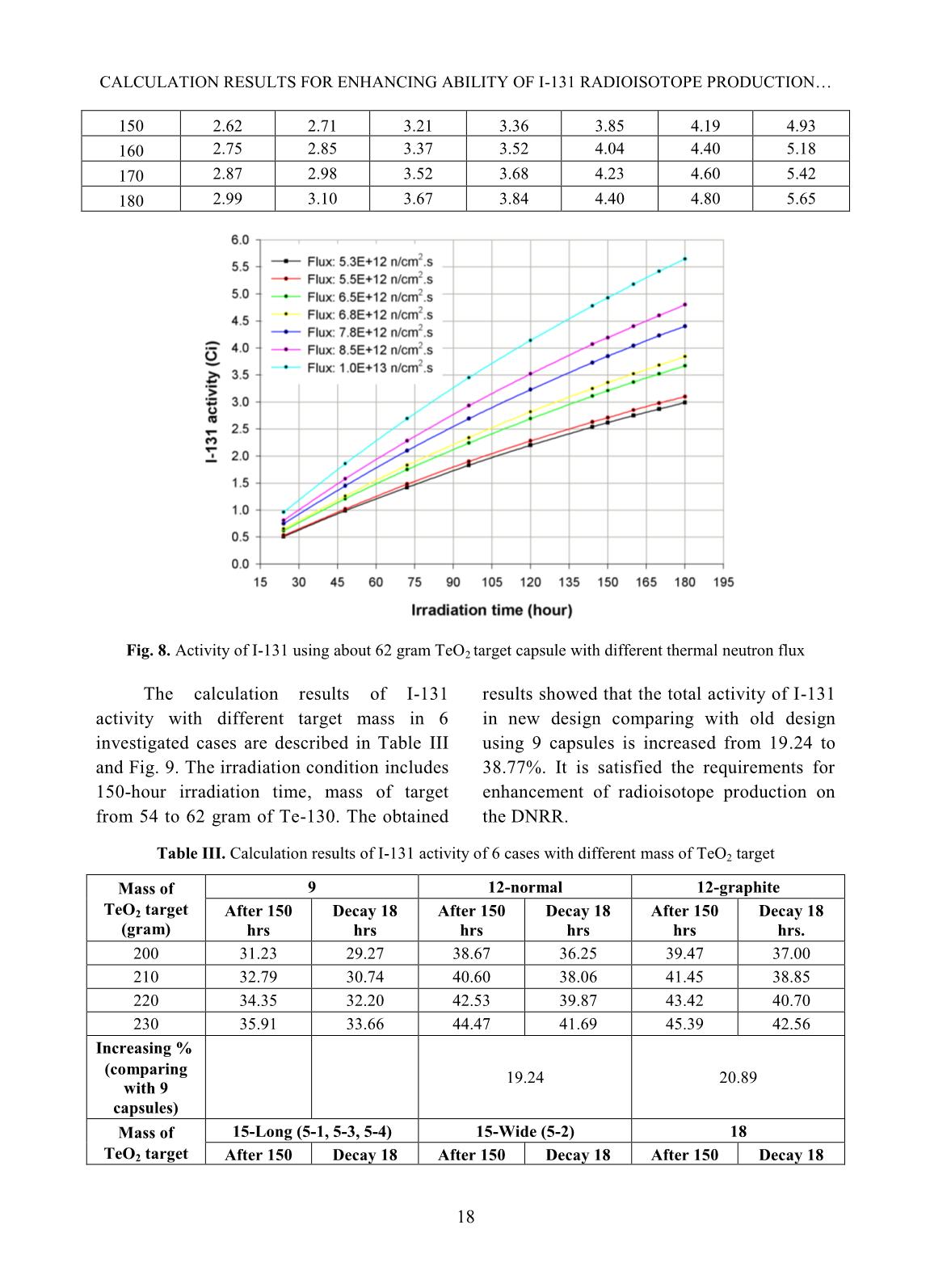
Trang 10
Tải về để xem bản đầy đủ
Tóm tắt nội dung tài liệu: Calculation results for enhancing ability of I-131 radioisotope production using tellurium dioxide target on the dalat nuclear research reactor
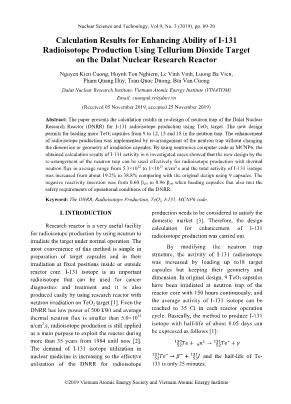
. The absolute neutron flux can be obtained as follows [5]: m efff m kw vP 1 106022.1 13 (2) where P is power of reactor (MW), v is the average number of neutrons released per fission, wf is effective energy released per fission (~ 193.7 MeV/fission to VVR-M2 LEU fuel type) and keff is the effective multiplication factor. Actually, getting data including neutron flux, reaction rates and effective multiplication factor from MCTAL file is easier than reading from output file of MCNP6 code. Reaction rates R are received in tally as: dEEER m n xmnx )()( (3) where Rmnx is reaction rate type x of nuclide n in cell m, micro cross section has unit barns. C. I-131 activity calculation after its irradiation and cooling The number density of I-131 can be calculated by equation [9]: CALCULATION RESULTS FOR ENHANCING ABILITY OF I-131 RADIOISOTOPE PRODUCTION 14 ( ) (4) (5) We can interfere that ( )( ( ) ) (6) With is micro cross section (barn); is neutron flux (n/cm 2 .s); is decay constant (s-1) and N is the number density of isotopes (atoms/cm 3 ). The equation can be solved by using numerical method or direct calculation after integrating the equation (6). Then, the number density of I-131 can be obtained as follows: ( )( ) ( ) (7) And tirr and tdecay are irradiation and decay time (hour), respectively. In the equation (7), we can separate as two parts with build-up process and decay time as follows: (8) + ( ( ) )( ( ) ( ) ) ( ) ( ) (9) The number density of Te-130 can be calculated by ( ) (10) With Avogadro number; ( )is mass of Tellurium in grams; ( ) is Atomic mass of Tellurium in grams. The activity A by Curie unit of I-131 can be determined by formula: (11) So the activity in each TeO2 target capsule can be determined through the calculation by applying equations from (4) to (11). I-131 activity can also be determined by using ORIGEN2.1 code with input data including irradiation time under specified neutron flux, cooling time and substituted capture cross section of Tellurium isotopes in activation material library. The thermal neutron flux and micro cross section of Tellurium isotope were estimated by MCNP6 code. III. CALCULATION RESULTS AND DISCUSSIONS A. Thermal neutron flux In the axial direction, the thermal neutron flux distribution of 1 cm/node at the original neutron trap without loading target compares with modified for loading 9 capsules (OLD) and the case having 6 irradiation NGUYEN KIEN CUONG et al. 15 channels (NEW). The profiles of thermal neutron flux in three cases have different in maximum point of 10% and in the average between OLD and NEW type. It is a disadvantage of thermal neutron flux when creating new irradiation channels around neutron trap. However in case adding only 2 new irradiation channels as in Fig. 4, the reducing of thermal neutron flux at neutron trap is not much and only under 5%. Fig. 5 depicts the profile of thermal neutron trap without loading capsules for radioisotope production. Fig. 5. Thermal neutron flux distribution at original neutron trap and modified for loading 9 capsules (OLD) and new irradiation positions (NEW) Basing on the obtained calculation results, the best distance in axial direction that has the highest thermal neutron flux is from 8 to 48 cm at bottom to the top direction. The height is equivalent to the length of 2 capsules with light water or graphite reflector at the top and the bottom. When loading TeO2 capsules at different irradiation positions inside the reactor core as in Table I, the average thermal neutron flux inside TeO2 target capsules is described in the Fig. 6. The maximum of average thermal neutron flux in the 9 capsules loaded is about 1.0×10 13 n/cm 2 .s while in other cases just of 8.8×10 12 , 8.3×10 12 and 8.0×10 12 with 15, 18 and 12 capsules loaded, respectively. The micro cross section in barn unit of Te- 130 isotope in thermal energy is about 1.53E-01 barns. The thermal neutron flux inside all capsules is high enough from 5.3×10 12 n/cm 2 .s to 1.0×10 13 n/cm 2 .s at top and middle position at irradiation channel. The detail axial distribution of each node of 0.78 cm/node inside 3 TeO2 target capsules from bottom to the top is depicted in the Fig. 7. CALCULATION RESULTS FOR ENHANCING ABILITY OF I-131 RADIOISOTOPE PRODUCTION 16 Fig. 6. The average thermal neutron flux in each capsule of 6 calculated cases Fig. 7. Relative thermal neutron flux of 3 capsules at each node in an irradiation channel from bottom to the top B. Negative reactivity and safety The negative reactivity insertion when loading capsules was also estimated by comparing with the core configuration without capsules. Detailed negative reactivity insertion for different loading number of capsules is depicted on the Table II with the difference of TeO2 mass in the capsules. The maximum of the negative reactivity insertion depends on the mass of loaded targets and it will be increased when increasing mass of Te-130. 5.0E+12 5.3E+12 5.5E+12 5.8E+12 6.0E+12 6.3E+12 6.5E+12 6.8E+12 7.0E+12 7.3E+12 7.5E+12 7.8E+12 8.0E+12 8.3E+12 8.5E+12 8.8E+12 9.0E+12 9.3E+12 9.5E+12 9.8E+12 1.0E+13 1.0E+13 1.1E+13 1 2 3 4 5 6 7 8 9 10 11 12 13 14 15 16 17 18 T h e rm al n e u tr o n f lu x (n /c m 2 .s ) Number of container 15-Long 15-Wide 9-old 12-Nor 12-Graph 18-Nor 10-Cont 15-Nor NGUYEN KIEN CUONG et al. 17 Table III. Negative reactivity insertion with different mass of Te-130 from 54 to 62 gram in each TeO2 target capsule Number of capsule Negative reactivity (cents) 9 (60.2 – 70.2) 12 normal (69.2 – 79.2) 12 with graphite (63.4 – 73.4) 15 long (83.8 – 93.8) 15 wide (81.4 – 91.4) 18 (86.5 – 96.5) The range of negative reactivity is met requirements for the reactor safety in normal operation. In thermal hydraulics, fuel cladding temperature is a parameter needed to be evaluated to assure the nuclear safety. As calculation results in the design of new LEU cores of the DNRR, the maximum temperature of the hottest channels in 2 core configurations is lower than permission temperature 103 0 C. So the rearrangement of neutron trap in 5 investigated cases will be also satisfied the limit of fuel cladding temperature after operating of 8 years. C. Activity of I-131 isotope in 6 investigated cases The main effects of irradiation for radioisotope production include thermal neutron flux, mass of target and irradiation time or decay time. With different neutron flux range from 5.3E+12 to 1.0E+13 n/cm 2 .s, irradiation time from 24 to 150 hours and the same target mass of 62 gram Te-130, calculation results are shown in Table II and Fig. 8. The increasing of neutron flux is proportional with I-131 activity in the scale 1 by 1. So the improvement of thermal neutron flux is a way to enhancement for radioisotope production. In case of the DNRR, the method to increase neutron flux is difficult because of its low power and design especially using fuel with low power density. If upgrading power of the DNRR from 0.5 MW to 0.6 or 0.8 MW the expected thermal neutron flux can be increased 16 to 35% respectively. Because the irradiation positions in axial direction of 3 capsules in each channel have different thermal neutron flux, the archived activity of I-131 after 150- hour irradiation of current 9 capsules has the ratio as 1.00:1.20:1.54 as top, bottom and middle positions of target. Table II. Calculation results of I-131 activity (Ci) at different thermal neutron flux and the mass of Te-130 is about 62 gram in a capsule Irradiation time (hr.) Activity of I-131 (Ci) with different thermal neutron flux (n/cm 2 .s) F=5.3E+12 F=5.5E+12 F=6.5E+12 F=6.8E+12 F=7.8E+12 F=8.5E+12 F=1.0E+13 24 0.51 0.53 0.62 0.65 0.75 0.81 0.96 48 0.99 1.02 1.21 1.26 1.45 1.58 1.86 72 1.42 1.48 1.75 1.83 2.10 2.28 2.69 96 1.83 1.90 2.24 2.34 2.69 2.93 3.45 120 2.20 2.28 2.69 2.82 3.23 3.52 4.14 144 2.54 2.63 3.11 3.25 3.73 4.07 4.78 CALCULATION RESULTS FOR ENHANCING ABILITY OF I-131 RADIOISOTOPE PRODUCTION 18 150 2.62 2.71 3.21 3.36 3.85 4.19 4.93 160 2.75 2.85 3.37 3.52 4.04 4.40 5.18 170 2.87 2.98 3.52 3.68 4.23 4.60 5.42 180 2.99 3.10 3.67 3.84 4.40 4.80 5.65 Fig. 8. Activity of I-131 using about 62 gram TeO2 target capsule with different thermal neutron flux The calculation results of I-131 activity with different target mass in 6 investigated cases are described in Table III and Fig. 9. The irradiation condition includes 150-hour irradiation time, mass of target from 54 to 62 gram of Te-130. The obtained results showed that the total activity of I-131 in new design comparing with old design using 9 capsules is increased from 19.24 to 38.77%. It is satisfied the requirements for enhancement of radioisotope production on the DNRR. Table III. Calculation results of I-131 activity of 6 cases with different mass of TeO2 target Mass of TeO2 target (gram) 9 12-normal 12-graphite After 150 hrs Decay 18 hrs After 150 hrs Decay 18 hrs After 150 hrs Decay 18 hrs. 200 31.23 29.27 38.67 36.25 39.47 37.00 210 32.79 30.74 40.60 38.06 41.45 38.85 220 34.35 32.20 42.53 39.87 43.42 40.70 230 35.91 33.66 44.47 41.69 45.39 42.56 Increasing % (comparing with 9 capsules) 19.24 20.89 Mass of TeO2 target 15-Long (5-1, 5-3, 5-4) 15-Wide (5-2) 18 After 150 Decay 18 After 150 Decay 18 After 150 Decay 18 NGUYEN KIEN CUONG et al. 19 (gram) hrs. hrs. hrs. hrs. hrs. hrs. 200 44.95 42.14 43.86 41.12 51.00 47.81 210 47.20 44.24 46.05 43.17 53.55 50.20 220 49.44 46.35 48.25 45.23 56.10 52.59 230 51.69 48.46 50.44 47.29 58.65 54.98 Increasing % (comparing with 9 capsules) 30.53 28.81 38.77 Mass of TeO2 target (gram) 10 (5-5) 13 (5-5) 15 (5-5) After 150 hrs. Decay 18 hrs. After 150 hrs. Decay 18 hrs. After 150 hrs. Decay 18 hrs. 200 37,18 34,85 41,26 38,68 45,32 42,48 210 39,03 36,59 43,32 40,61 47,58 44,61 220 40,89 38,33 45,39 42,55 49,85 46,73 230 42,75 40,08 47,45 44,48 52,11 48,85 Increasing% (comparing with 9 capsules) 16.00 24.32 31.10 Fig. 9. Total activity of I-131 with different TeO2 mass and under 150-hour irradiation In 6 new cases considered, the simplest way is rearrangement of neutron trap with an option to create 2 irradiation channels by replacing the beryllium rods at cell 5-6 and 9- 6 to load 6 capsules more. The new core configuration still keeps the neutron trap, 92 LEU fuel assemblies and 10 beryllium rods. And this core configuration was confirmed the safety by evaluating the maximum fuel cladding temperature by PLTEMP4.2 code [10] at hottest fuel assembly located at cell 4- 5. Following the calculation results, under 27 o C of water at core inlet, the maximum temperature is still lower than 88 o C and meets requirements for operating limit condition of the VVR-M2 LEU fuel type of the DNRR. CALCULATION RESULTS FOR ENHANCING ABILITY OF I-131 RADIOISOTOPE PRODUCTION 20 IV. CONCLUSIONS AND REMARKS The calculation about the enhancement radioisotope production for I-131 by rearrangement of neutron trap to loading more target capsules using MCNP6 code were conducted. In general, 6 cases for enhancement of I-131 radioisotope production on the DNRR can be carried out easily and meet the safety requirements in reactor operation. Comparing with old design using 9 containers, the total activity of I-131 isotope was increased from about 19.2% to 38.8% with loading TeO2 target mass increasing from 25% to 50%. The rearrangement of beryllium rods around neutron trap at cell 5-6 and 9-6 to create 2 new irradiation channels is highly considered because of safety, easy implementation and effectiveness in production of I-131. The design calculation is a very important step before carrying out testing a reactor operating cycle with new design for changing neutron trap to satisfy safety of the normal operation condition of the DNRR. The increasing I-131 product and operation time as well as using economical fuel are the main purposes in effective utilizations and applications of the DNRR. The calculation results can also be used as reference for the new research reactor in design of radioisotope production especially of I-131 isotope. ACKNOWLEDGEMENT The authors would like to express special thanks for the helpful discussions and willing carrying out experiments on the DNRR of the staff in Reactor Physics and Engineering Department, Reactor Center. We also appreciate the financial support, valuable helping of Ministry of Science and Technology and VINATOM for implementation of the research project: “Study on effective, economic in using fuel and enhancement of radioisotope production on the Dalat Nuclear Research Reactor” with grant number ĐTCB.10/191 VNCHN. REFERENCES [1]. International Atomic Energy Agency, Manual for reactor produced radioisotopes, IAEA TECDOC-1340. IAEA, Vienna, Austria; 2003. p. 121-4. [2]. Nuclear Reesearch Institute, Safety Analysis Report for the DNRR, version 2012. [3]. Nguyen Kien Cuong et al., “Some enhancement plans for radioisotope production at neutron trap of the reactor core using LEU fuel”, the 10th National Conference on Nuclear Science and Technology, Vung-tau City, 2013. [4]. https://wwwndc.jaea.go.jp/j40fig/jpeg/te130_f 1.jpg [5]. X-5 Monte Carlo Team, MCNP – A General Monte Carlo N-Particle Transport Code, Version 5, LAUR-03-1987, Los Alamos National Laboratory, Los Alamos, New Mexico (2003). [6]. A.G. Croff, A User Manual for the ORIGEN2 Computer Code, Oak Ridge NL, 1980. [7]. LUONG Ba Vien, LE VinhVinh, HUYNH Ton Nghiem, NGUYEN Kien Cuong, “Design Analyses for full core conversion of the Dalat Nuclear Research Reactor”, Nuclear Science and Technology,Vol. 4. No. 1 (2014), pp. 10- 25, Vietnam Atomic Energy Association, August, 2014. [8]. https://www.oecd- nea.org/dbdata/jeff/jeff33/#_jeff- 33_downloads [9]. Abdessamad Didi, Ahmed Dadouch, Hassane El Bekkouki, “Feasibility study for Production of Iodine-131 using dioxide of Tellurium- 130”, International Journal of Pharmaceutical Sciences 2016, Vol. 8, Issue 11, 327-331. [10]. P. Olson and M. Kalimullah, “A User’s Guide to the PLTEMP/ANL Code, Version 4.2”, Nuclear Engineering Division, Argonne National Laboratory, USA, July 7, 2015.
File đính kèm:
calculation_results_for_enhancing_ability_of_i_131_radioisot.pdf