Integration of solar pv systems into grid: Impact assessment and solutions
The integration of Renewable Energy Resources (RES) or PV systems into
grid, with the intermittent characteristics can have several impacts on the network
operation such as stability, protection and challenges for managing Theses impacts
are more complicated for an islanded grid or weak grid. To facilitate the integration of
renewable energies into the grid, a concept of smart-grid is used. The smart grid uses
digital technology to improve reliability, flexibility, and efficiency (both economically
and energetically) of the electric system. This paper presents impacts provided by PV
systems integration into grid: voltage variations, frequency variation, voltage
unbalance Several solutions in order to reduce these impacts, to maximize the
ancillary services contributed by PV systems are proposed via different projects.
Intelligent control and energy management are developed in order to minimize
operation cost and to maximize the RES penetration rate into grid.
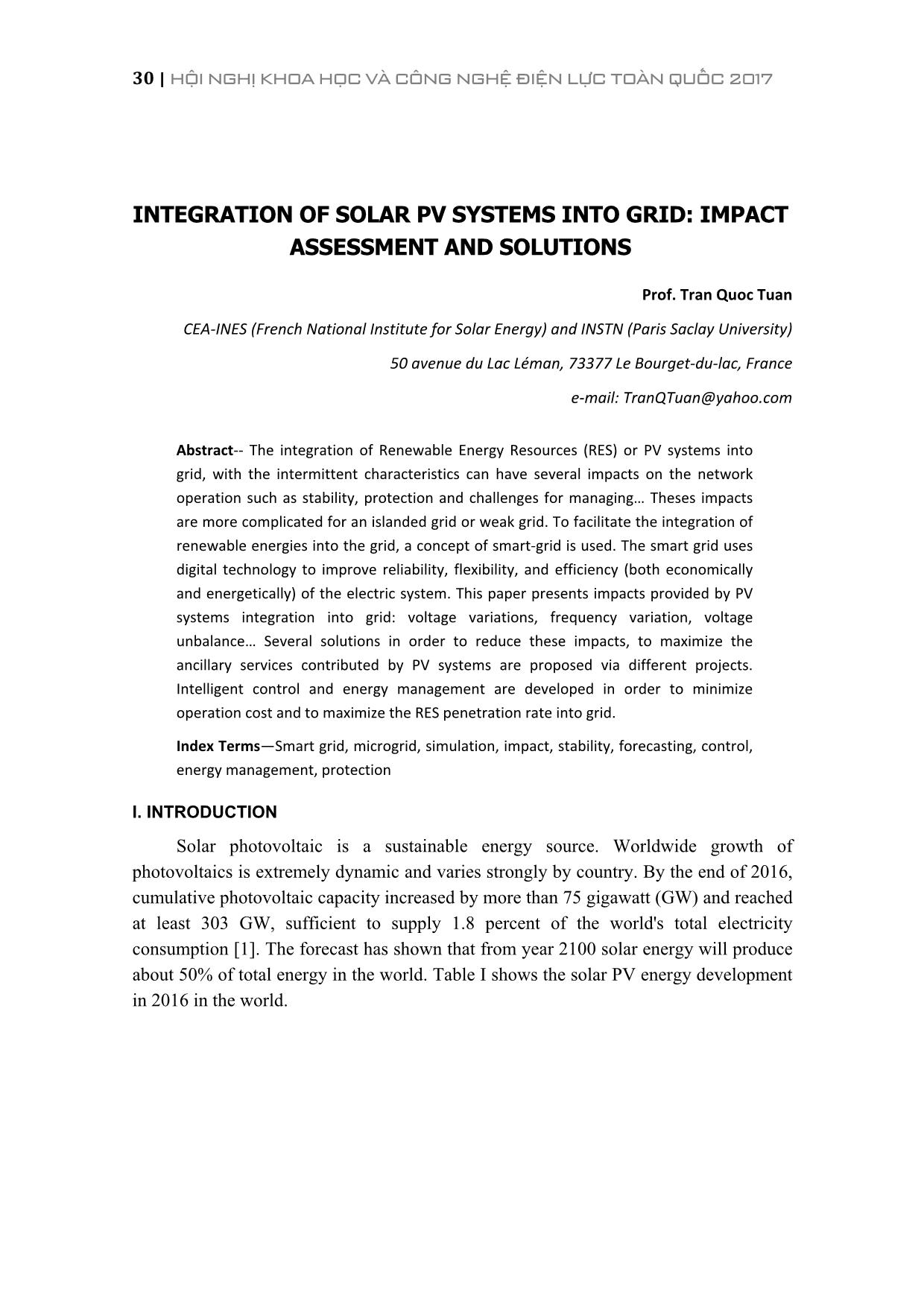
Trang 1
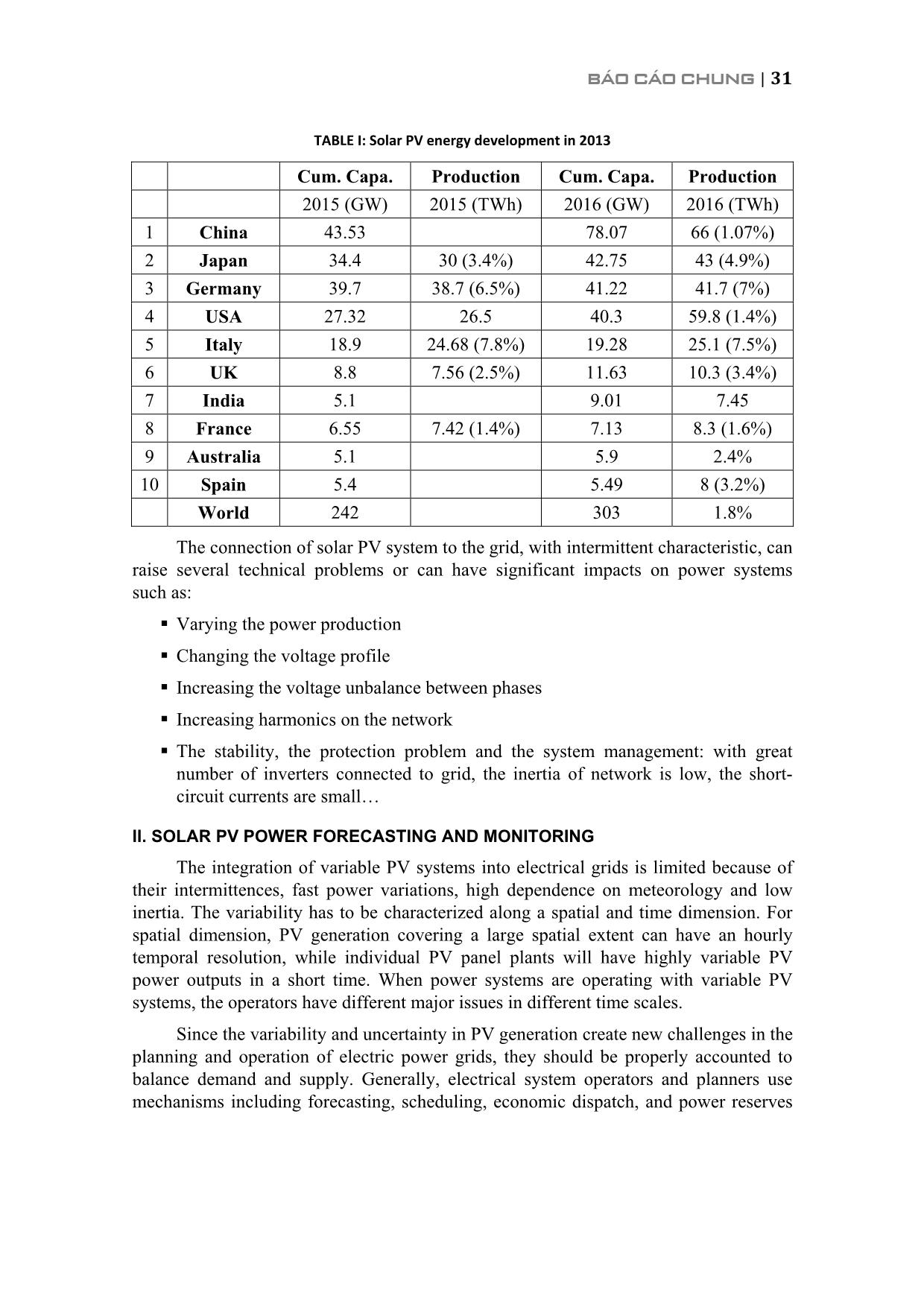
Trang 2
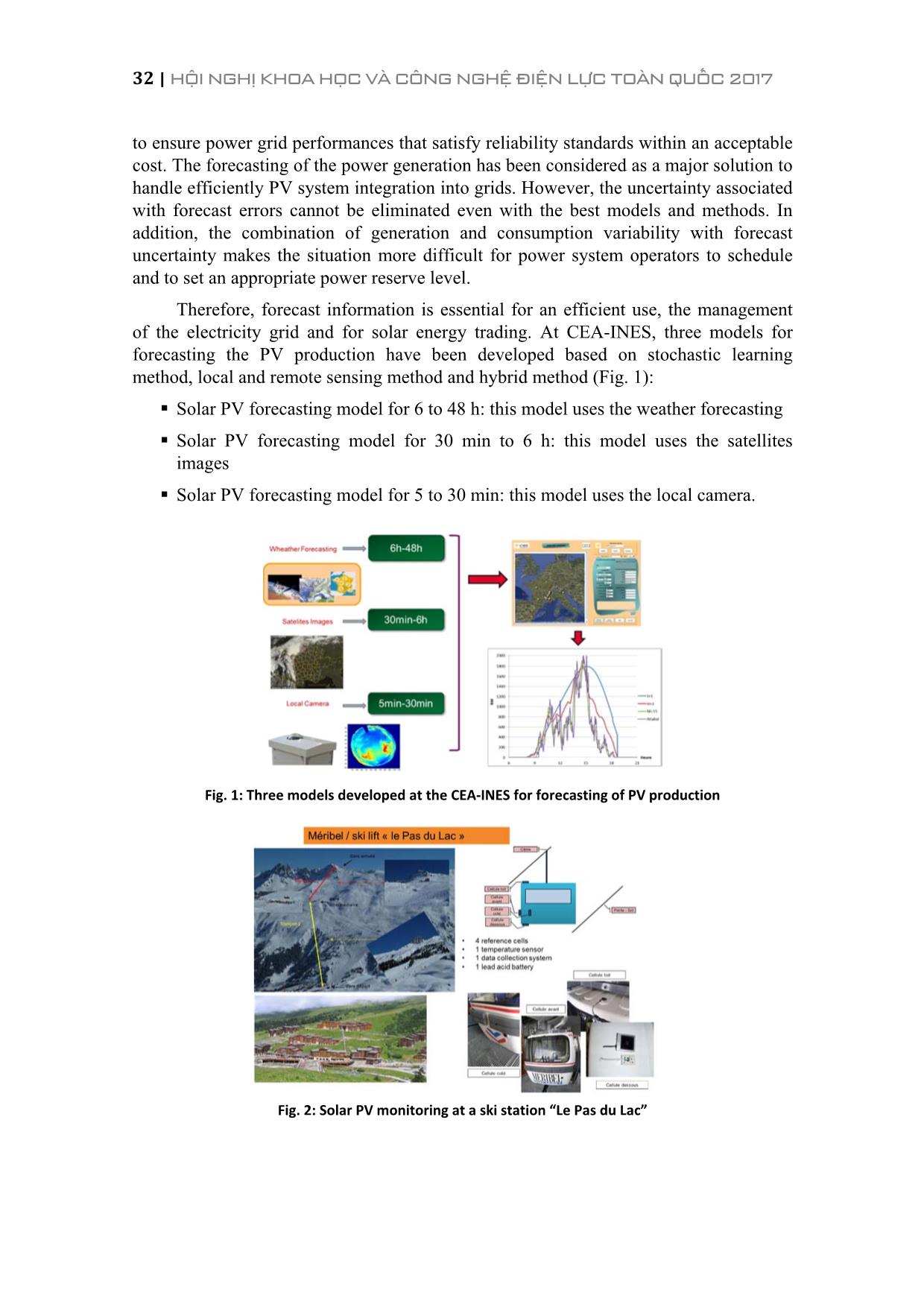
Trang 3
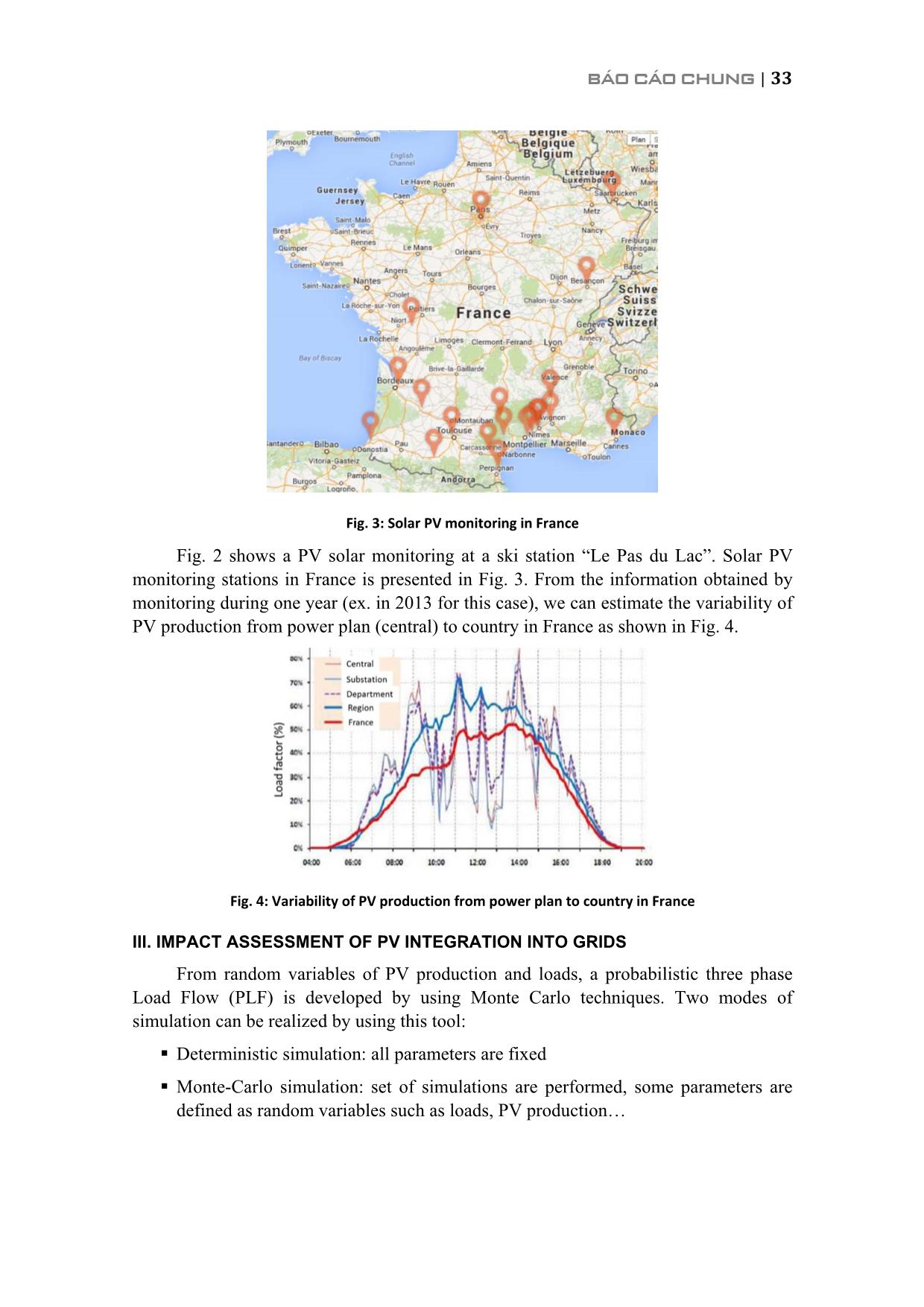
Trang 4
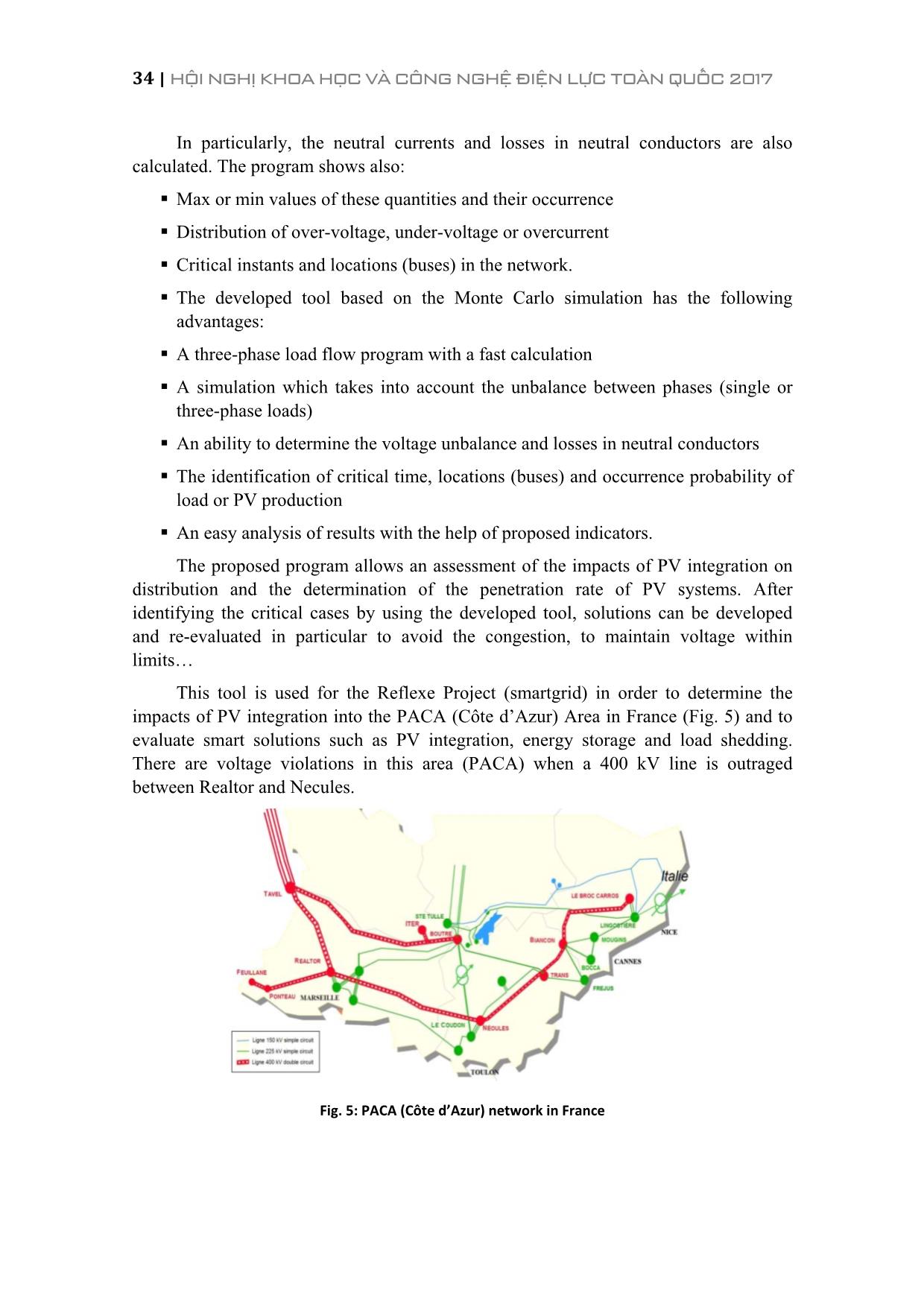
Trang 5
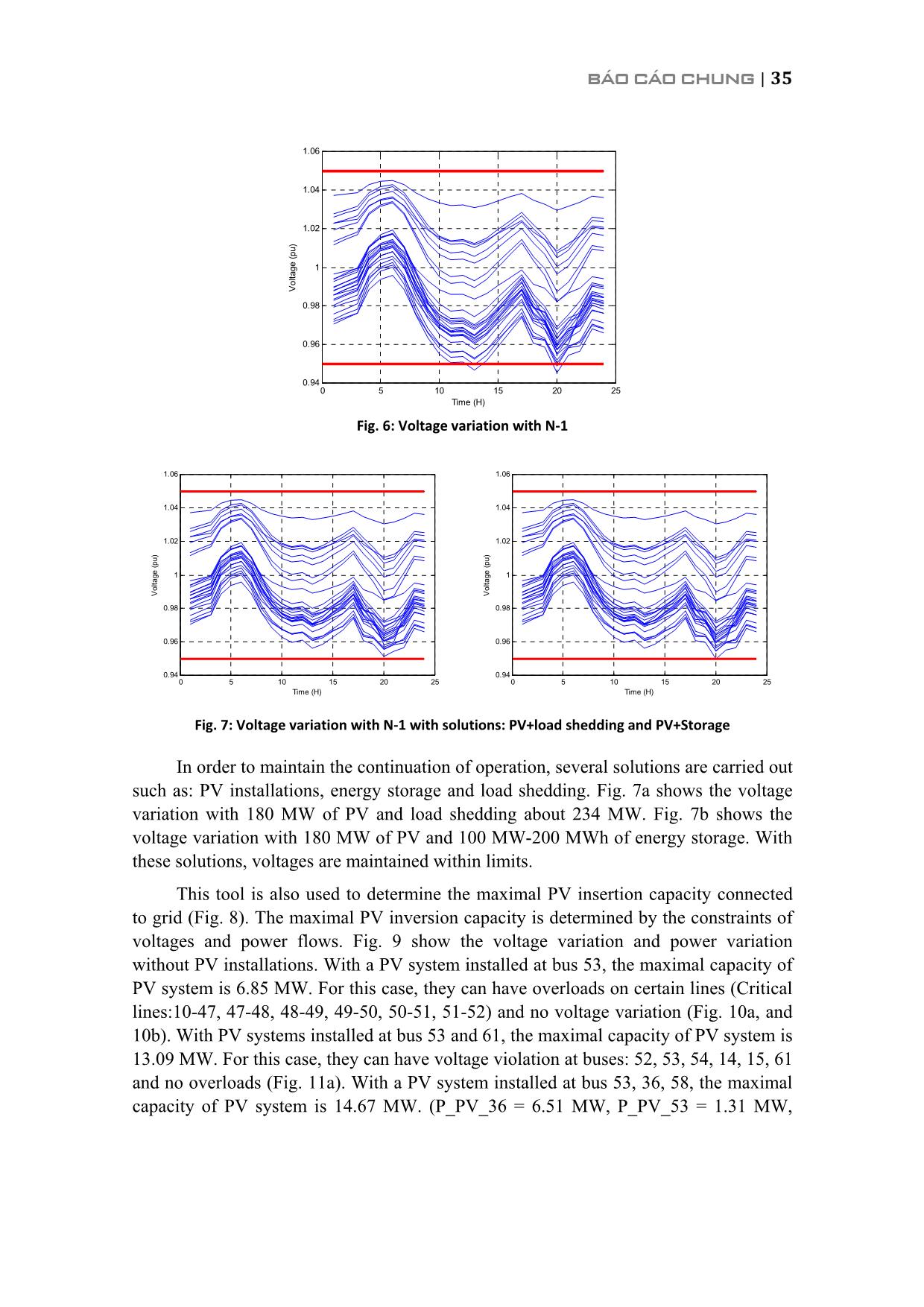
Trang 6
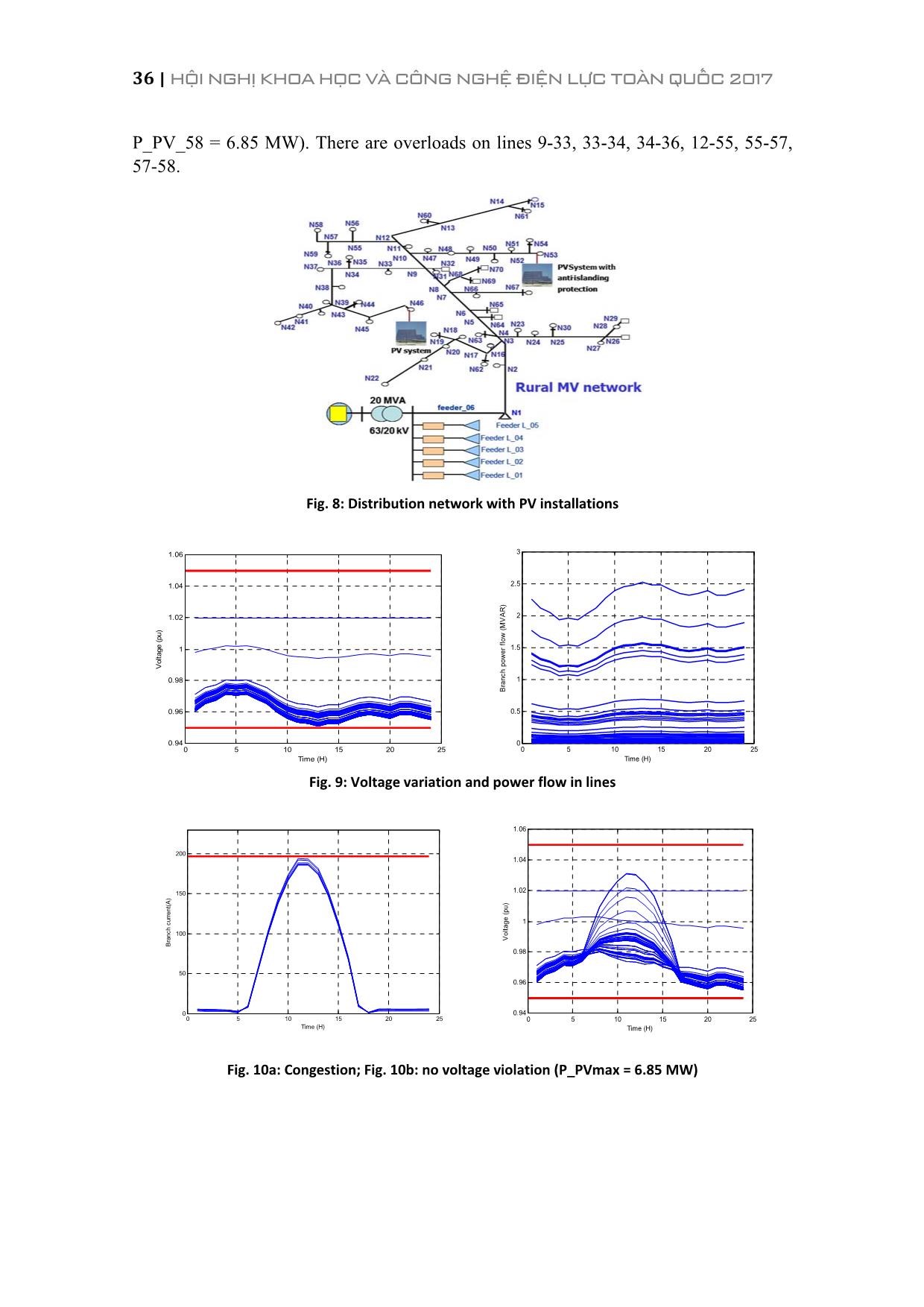
Trang 7
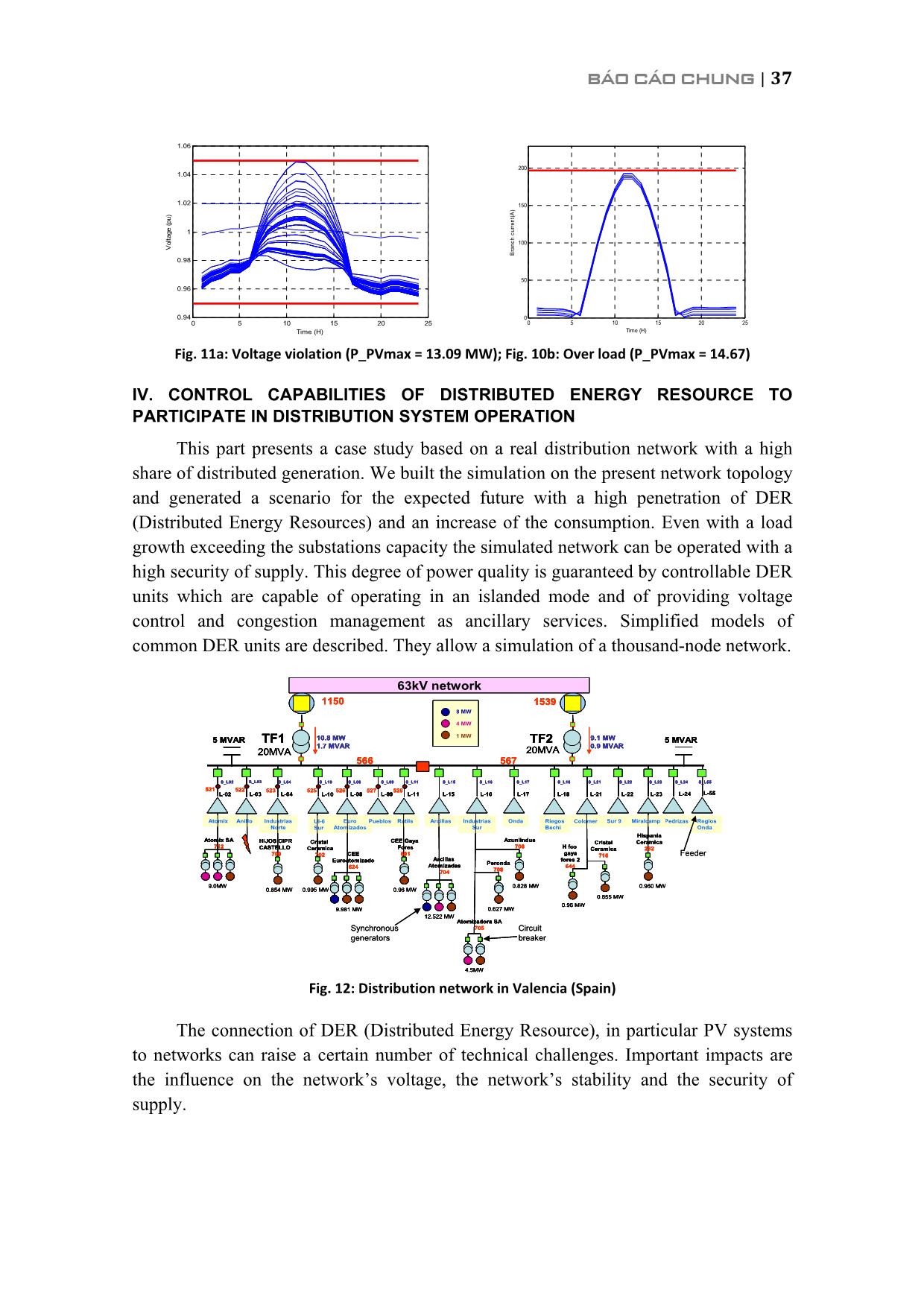
Trang 8
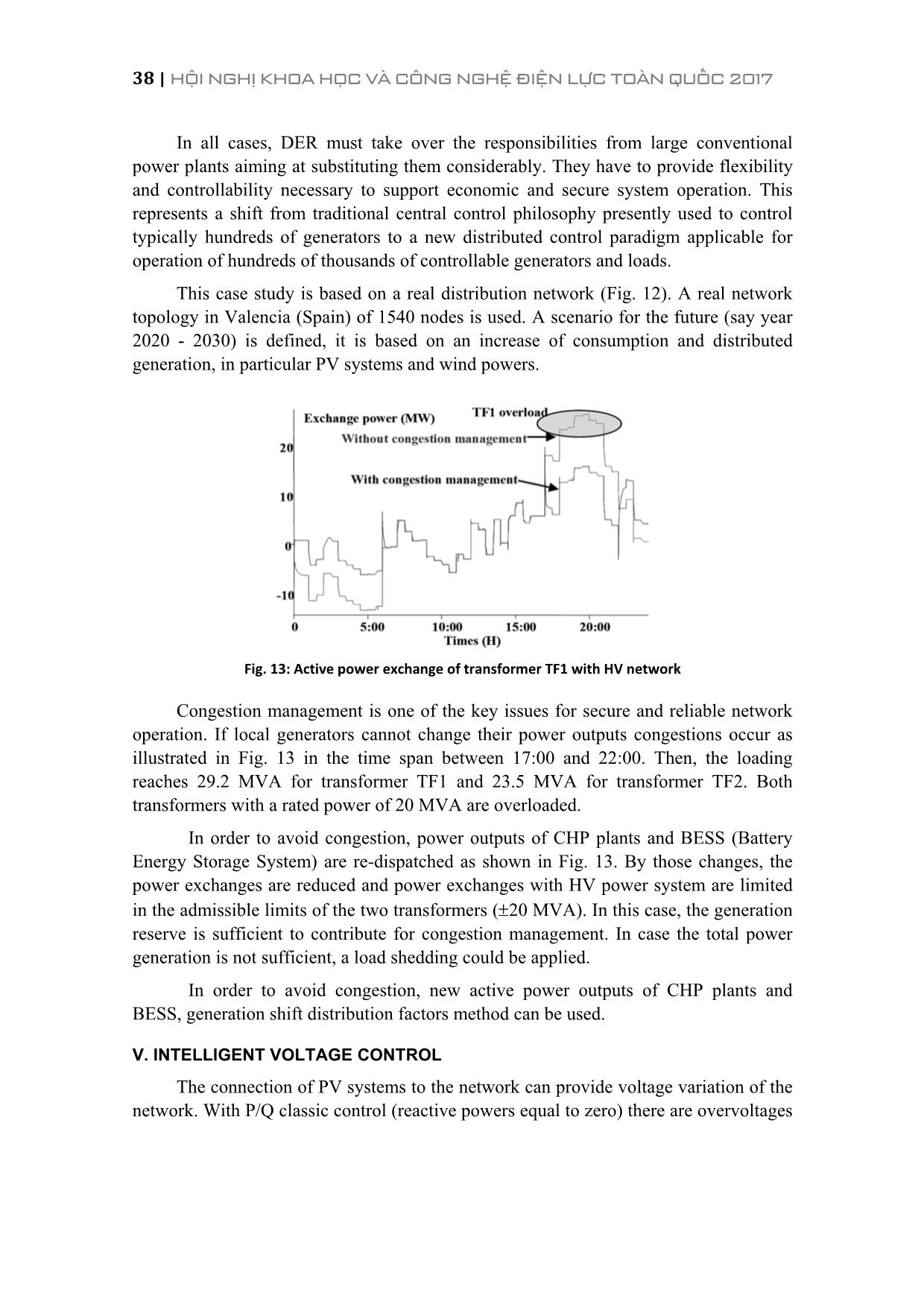
Trang 9
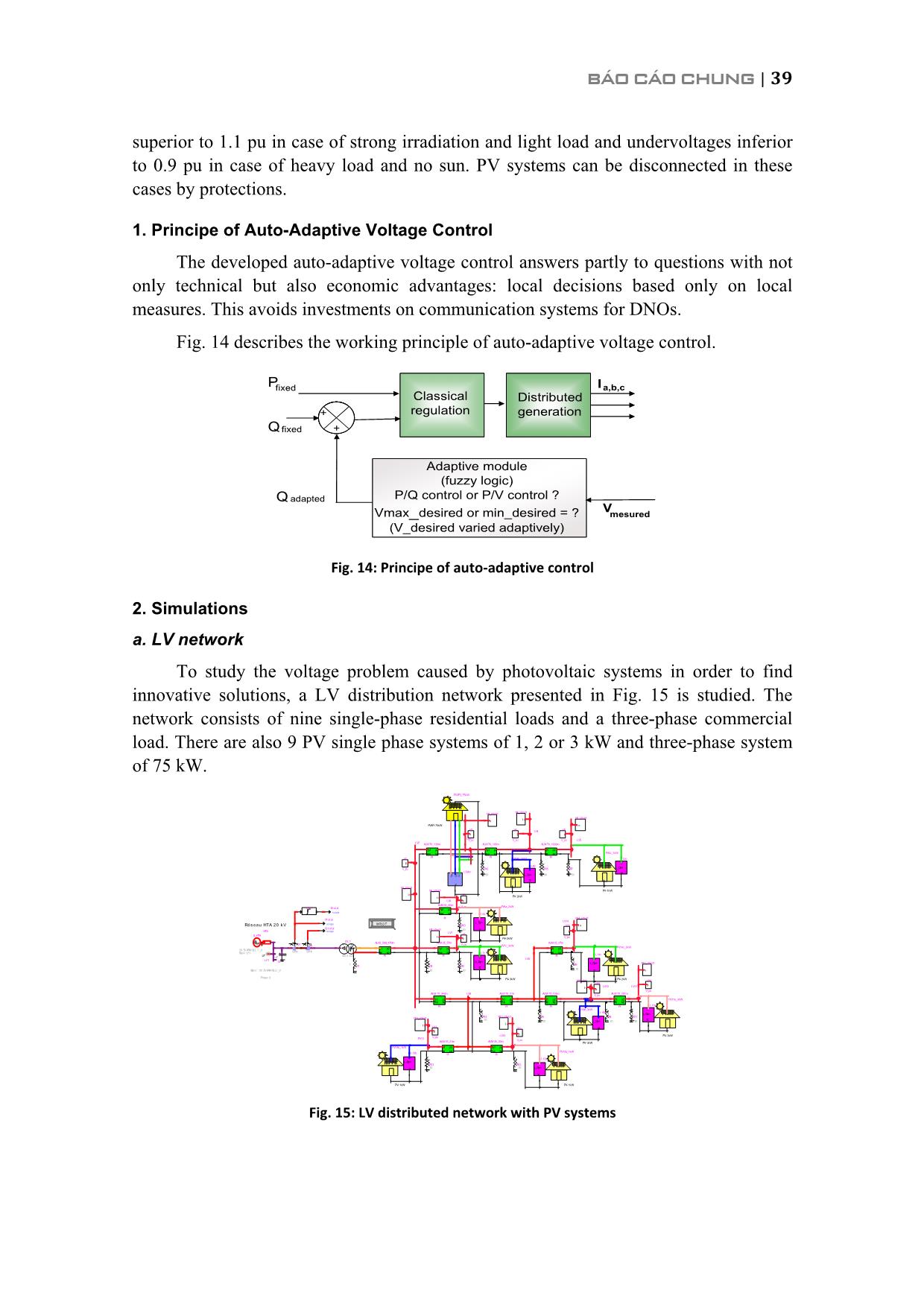
Trang 10
Tải về để xem bản đầy đủ
Tóm tắt nội dung tài liệu: Integration of solar pv systems into grid: Impact assessment and solutions
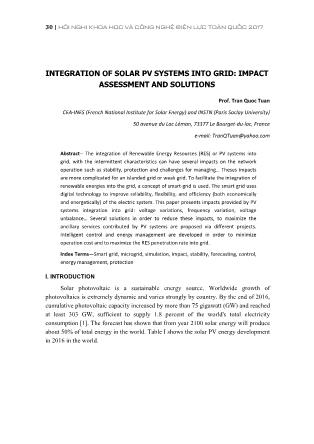
ehaviour of PV systems in a short circuit condition, a LV distribution network is used (Fig. 27). This network is powered by a 400 kVA (20/0.4 kV) distribution transformer. This network comprises 2 feeders with underground cables of 240 mm2. Each feeder has a FD 400 A fuse. The distance between the customers is 10 or 20 m. The the customers are well distributed among the three phases. Each connection has an AD 90 A fuse. It is assumed that there are three 3 kWp PV systems connected on three phases to nodes 21, 23 and 24, respectively. The anti-islanding protection of PV systems is based on the voltage and frequency criterion according to DIN VDE 0126-1-1. Different types of short circuits (single-phase, phase-phase, three-phase) are created at the different points of the network on the two feeders. The behaviours of PV inverters in the event of 46 | HỘI NGHỊ KHOA HỌC VÀ CÔNG NGHỆ ĐIỆN LỰC TOÀN QUỐC 2017 a short circuit are observed. In this section, we present only the behaviors of PV inverters in the case of a single-phase short-circuit. Fig. 27: LV distributed network with PV systems and protection systems Fig. 28: Behaviour of PV systems in fault phase and non-fault phase during a sing phase short circuit BÁO CÁO CHUNG | 47 A single-phase short-circuit appears at t = 1.0 s on phase a at node 7. The short- circuit current seen by the fuse FD1 reaches 7 kA (Fig 28a). This fuse melts at t = 1.41 s. During the short circuit, the voltage of the PV inverter connected to the faulty phase (at node 21) decreases to 0.52 pu (Fig. 28c). This PV inverter is disconnected 200 ms after the short circuit (t = 1.2 s). During the short circuit, the current of this inverter increases slightly but does not exceed 110% (Fig. 28d). Fig. 28e and Fig. 28f show the power and voltage variations of the PV inverter connected to the non-fault phase (phase b to node N23). This inverter maintains the connection during and after the fault. This is identical with the PV inverter connected on phase c to node N24. We thus show the support capacity to disturbance for the inverters on the non- fault phases. Future power systems with high penetration of photovoltaic (PV) systems could be severely affected in a power quality disturbance, generation loss and stability problem if several of PV systems are tripping. Among power quality disturbances, voltage sag is considered as the most serious which can cause the disconnection of a significant amount of PV systems. These disconnections are sometimes unnecessary, particularly when the fault is not situated on the same feeder of PV systems (adjacent feeder). Therefore, this part focuses on voltage sags caused by short circuits. This work is to study behaviors of grid-connected PV systems facing voltage sags by taking into account the real urban network (MV network) protections. From simulations, unnecessary trip cases of PV systems are then identified. Finally, a solution by using the voltage-times characteristic for PV system is proposed (Fig. 29). This solution permits to avoid unnecessary trip of PV systems in case of a short circuit on the adjacent feeder, outside of PV zones. Nevertheless, in case of a short circuit in their zone, PV systems are disconnected. A verification of the system operation with proposed solution is also carried out. Fig. 29: PV voltage in comparison with the voltage-time characteristic for a fault on LV adjacent feeder (outside of the PV zone) 48 | HỘI NGHỊ KHOA HỌC VÀ CÔNG NGHỆ ĐIỆN LỰC TOÀN QUỐC 2017 VIII. STRATEGIES FOR BESS MANAGEMENT IN PHOTOVOLTAIC APPLICATIONS High capital investment cost for batteries constitute a major obstacle to the widespread deployment of battery energy storage systems (BESSs) as a tool to support the integration of renewable energy sources (RES) into the electricity grid. BESSs are already operated in commercial MW-scaled photovoltaic (PV) installations to facilitate power production levelling and participation in auxiliary services, such as frequency and voltage control. The optimization of dispatch schedules has been employed in the past to aid the sizing of BESS and drive down capital expenditures by limiting the installed battery capacity to the minimum required for the application. In this work, an optimized operation strategy is used to reduce replacement costs for the batteries by adapting the state-of-charge and power solicitation to achieve minimized aging. To this end, a simplified model for both calendar and cycle aging of Li-ion batteries is integrated into a mixed-integer linear programming algorithm. Results from a simulation using typical specifications for a BESS operated in a PV power plant in French overseas regions are presented and discussed. Fig. 30: Architecture of photovoltaic power plant with two parallel battery energy storage systems. Fig. 31: Plant production schedule, PV generation and BESS solicitation resulting from optimization. Top: without aging cost, bottom: with aging cost (average aging approach) BÁO CÁO CHUNG | 49 Fig. 32: One-day production schedule of two ESSs and a PV power plant in a VPP, as well as net market bids in the day-ahead energy (Market) and tertiary reserve market (Res). Top: current German market rules, bottom: quarter-hourly energy market contracts and relaxed minimal reserve amount constraint. IX. STABILITY IN AN ISLAND NETWORK WITH HIGH RES INTEGRATION In a context where renewable energies from both wind and solar origin have low predictability, low controllability and strong variability, their massive integration into power systems may cause instabilities for these grids. The use of storage energy systems is a promising solution for solving such problems. In the present part, we focus on flywheel energy storage system (FESS) associated to photovoltaic power generation (PV). The FESS is then connected to a grid simulated by real time simulator in order to test its ability for grid ancillary service: voltage, frequency regulation and PV power smoothing. According to the inertia of the grid, the flywheel system is more or less able to smooth the frequency, the voltage and the PV production. Two application of FESS are studied: PV power smoothing with three control methods (Fig. 33), Frequency control (Fig. 34). Results obtained for these cases shows efficiency evaluation of the proposed solutions. This is in framework of smart grid in Corsia island (France). 50 | HỘI NGHỊ KHOA HỌC VÀ CÔNG NGHỆ ĐIỆN LỰC TOÀN QUỐC 2017 Fig. 33: Results of the PV smoothing with different control methods. PV power and PV variation are in red solid line. Blue solid lines represent data from the 2-D supervisor, dashed green lines data from the 3-D supervisor with rate limiter and dotted black lines data from the 3-D supervisor with LPF. From the top graph to the bottom graph: the first graph gives the smoothed powers injected to the grid, PV power (red) and flywheel powers; the second the speeds of the flywheel; the 3rd energies lost by the flywheel and the last graph the power variations of each control method compared with the PV power variation (red) Fig. 34: Frequency variation with and without frequency control (a) and Flywheel Power (b) in case of change of PV production This is in framework of smart grid in Guadeloupe island, France (Fig. 35). The high penetration of RES into a isolated grid can provide the stability problem. Fig. 36 shows the frequency variations in Guadeloupe with the penetration rate of RES (PV) about 2, 32, 50 and 72%, respectivelly in case of short-circuit without or with the intelligent protection strategies. Without solution this grid is instable and the maximal penetration rate of RES (PV) in this grid is limited about 30%. In order to increase the penetration rate of RES (PV), several intelligent solutions for control and protection strategies are proposed. With the proposed solutions this grid is always stable for different penetration rates, even with 72% of RES (PV) (Figs 36 to 38). 0 250 500 750 1000 1250 1500 1750 2000 2250 2500 2750 3000 -5000 0 5000 10000 15000 Po w er (W ) 0 250 500 750 1000 1250 1500 1750 2000 2250 2500 2750 3000 0 2500 5000 6000 Sp ee d (R PM ) 0 250 500 750 1000 1250 1500 1750 2000 2250 2500 2750 3000 -500 -350 -1000 E f w (W h) 0 250 500 750 1000 1250 1500 1750 2000 2250 2500 2750 3000 -60 -30 0 30 60 Po w er va ria tio n (% ) Time (s) 95 100 105 110 115 120 125 130 49 49.25 49,5 49.75 50 50.25 50.5 50,75 Time (s) Fr eq ue nc y (H z) Frequency without control (Hz) Frequency with control (Hz) 95 100 105 110 115 120 125 130 -5 000 -4000 -2500 0 2500 4500 Time (s) Fl yw he el P ow er (W ) BÁO CÁO CHUNG | 51 Fig. 35: Isolated grid of Guadeloupe Fig. 36: Frequency variation for 3, 32, 50 and 72% of RES (PV) penetration without and with proposed solutions Fig. 37: Voltage variation for 3, 32, 50 and 72% of RES (PV) penetration without and with proposed solutions 52 | HỘI NGHỊ KHOA HỌC VÀ CÔNG NGHỆ ĐIỆN LỰC TOÀN QUỐC 2017 Fig. 38: Rotor angle variation for 3, 32, 50 and 72% of RES (PV) penetration without and with proposed solutions X. CONCLUSIONS The integration of variable RES (PV systems) into electrical grids is limited because of their intermittences, fast power variations, highly dependence on meteorology and low inertia. In this paper, the forecasting, the monitoring of solar PV power have been investigated. Impacts of PV system integration in grid operation have been also analyzed. Several solutions in order to reduce these impacts, to maximize the ancillary services contributed by PV systems have been proposed via different projects. Intelligent control and energy management have been developed in order to minimize operation cost and to maximize the RES penetration rate into grid. REFERENCES [1] https://en.wikipedia.org/wiki/Solar_power_by_country [2] T. Tran-Quoc, X. Le Pivert, J. Merten, “Analysis of Photovoltaic Generation Impacts on Distribution Networks”; Conférence PVSEC - The 27th European Photovoltaic Solar Energy Conference and Exhibition, 24 - 28th September 2012, Frankfurt (DE). [3] Cédric Abbezzot, Tuan Tran Quoc, “Flywheel Energy Storage system used in a Photovoltaic Power Generation System”, 3rd International Conference on Energy Process Engineering (ICEPE 2013), Franfurk, Germany, 4-6th june, 2013. [4] NA Luu, T. Tran-Quoc, “Optimal energy management for an island microgrid by using Dynamic programming method”; Conference IEEE/Powertech, 29th June – 2nd july 2015, Eindhoven, Holand. BÁO CÁO CHUNG | 53 [5] E. Krüger and Q. T. Tran, "Minimal aging operating strategies for battery energy storage systems in photovoltaic applications", IEEE-ISGT, Innovative Smart Grid Technologies Conference, 9-12 October 2016, Ljubljana, Slovenia. [6] Q. T. Tran, NA Luu, "Optimal Energy Management Strategies of Microgrids", The 2016 IEEE Symposium– Special Session: Intelligent Management of Micro Grids and Buildings (IEEE SSCI - CIASG 2016) – 6-9 december 2016 Athens, Geece. Ph.D. thesis T1. Guillaume RAMI, "Adaptive voltage control for distributed generations connected to distribution networks", PhD thesis prepared at the Grenoble-INP, Defended on 9 November 2006. T2. Thanh Luong LE “Detection of instability in grid with high RES penetration”, PhD thesis prepared at the Grenoble-INP, Defended on 22 January 2008. T3. Thi Minh Chau LE, "Coupling Photovoltaic inverters to the network, aspects of control and support capacity for disturbances", PhD thesis prepared at the Grenoble-INP, Defended on 25 January 2012. T4. Van Linh NGUYEN, "Coupling photovoltaic systems and electric vehicles to the grid: Problems and solutions"; PhD Thesis prepared at the Grenoble-INP and CEA-INES, Defended on 1st October 2014. T5. Cedric ABBEZZOT, "Flywheel energy storage system coupled to the photovoltaic generator and controlled by a real-time simulator"; PhD Thesis prepared at the CEA-INES, Defended on 15 December 2014. T6. Ngoc An LUU, "Strategies of control and management for microgrids", PhD Thesis prepared at the CEA-INES and G-INP, Defended on 18 December 2014. T7. Eiko KRUGER, "Development of algorithms for optimal management of energy storage systems based on adaptive models", PhD Thesis prepared at the CEA-INES, Defended on 21 November 2016. T8. GABIN A. KOUCOI, "Energy Management in PV/Diesel hybrid system for isolated and rural zones: optimization and experimentation”, PhD Thesis prepared at the CEA-INES and University of Burkina Faso, Defended on 28 February 2017. T9. Elvira AMICARELLI, "Management strategy for power grids with a high rate of distributed renewable production", PhD Thesis prepared at the CEA-INES, Defended on 16 October 2017. T10. Hélène CLEMOT, "Strategies for optimal management of marine wave resources", PhD Thesis prepared at the CEA-INES and ECN, Defended in 2017. T11. Tung Lam NGUYEN, "Smart control strategies in Microgrids with Multi Agent Systems", PhD Thesis prepared at the CEA-INES and G-INP. T12. Karla SOUSA, "Stability in large network with high RES penetration”. T13. Laurène PARENT, "Primary and secondary reserve in large network with high RES penetration". T14. Anthony ROY, "Management of an island grid in ocean (Marine+PV+wind+storage)". T15. Tran The HOANG, "Smart protection strategies in Microgrid". T16. Tai LE, "New Architecture of PV power plants". T17. A. Arief, “Self-consumption and ancillary services”. T18. I. Karray, “Stability and HVDC solution”. 54 | HỘI NGHỊ KHOA HỌC VÀ CÔNG NGHỆ ĐIỆN LỰC TOÀN QUỐC 2017 Several Projects [P1] GREENLYS: Smart-grid demonstration. [P2] REFLEXE: Smart-grid demonstration at the south-east of France. [P3] PARADISE (ANR PROGELEC Project ID: ANR-13-PRGE-0007): high penetration of renewable energy resources into grid with DS. [P4] ERIGRID (H2020, European project ID: 654113) European Research Infrastructure supporting Smart Grid Systems Technology Development, Validation and Roll Out. [P5] SEAS (Call 7 ITEA2, European project No. 1204) Smart Energy Aware Systems. [P6] UNITED-GRID (H2020, European Project ID: 314175): Autonomous Management System Developed for Building and District Levels. [P7] M2M GRID (ERA-NET, European Project ID: 82136): From micro to Mega GRID: Integration approach for the new generation of smart grids. [P8] PPInterop (Carnot project): Interoperability between two platforms: PRISME-PREDIS. [P9] FENIX (European project) Virtual power plants. [P10] DERri (European project No.: 228449) Tools and methodologies to perform steady-state, dynamic and real-time simulation of DER and power systems –Hardware Interfaces.
File đính kèm:
integration_of_solar_pv_systems_into_grid_impact_assessment.pdf