Effect of temperature on dielectric, ferroelectric, and piezoelectric properties of knlns - bnkz ceramics
Due to their ability of interconverting mechanical energy to electrical energy and
vice versa, piezoelectric materials are indispensable parts in all ultrasonic devices (Jaffe
et al., 1971; Uchino, 2000). At present, (K0.5Na0.5)NbO3 (KNN)-based lead-free ceramic
materials are attracting a lot of attention (Tan et al., 2018; Zhai et al., 2019; Zhang et al.,
2020) because they not only have high piezoelectric properties and high Curie
temperatures (Saito et al., 2004; Zang et al., 2006), but they are also environmentally
friendly. Therefore, KNN-based lead-free piezoelectric ceramics are the best replacement
for materials based on toxic lead in the ultrasonic field (Uchino, 2000).
In recent years, there has been an increased demand for powerful ultrasonic
transducers for large-scale industrial applications. Transducers for such purposes require
high-quality piezoceramic materials because the temperature of the transducer will
increase during operation, affecting the stability of the piezoelectric properties and
increasing the aging of the material (Uchino, 2000). If the quality of the material is poor,
the piezoelectric properties of the material can be drastically weakened, which affects the
efficiency of the transducer. Consequently, consideration should be given to the
temperature stability of the piezoelectric properties of fabricated materials in practical
applications.
This paper presents the results of the fabrication and study on the temperature
dependence of the physical properties of 0.96(K0.48Na0.48Li0.04)(Nb0.95Sb0.05)O3-
0.04Bi0.5(Na0.82K0.18)0.5ZrO3 lead-free piezoelectric ceramics to determine the stable
temperature range of their ferroelectric and piezoelectric properties.
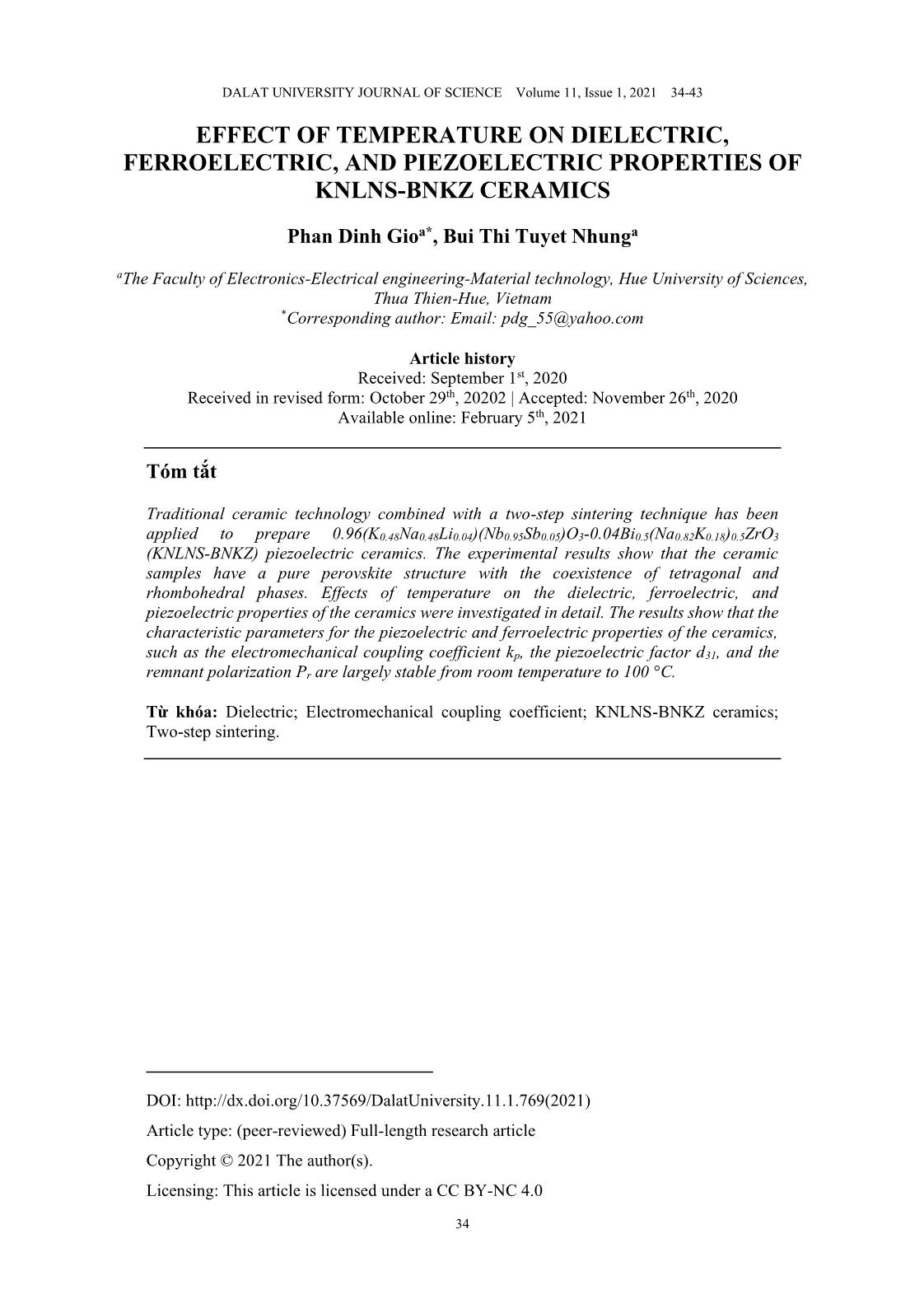
Trang 1
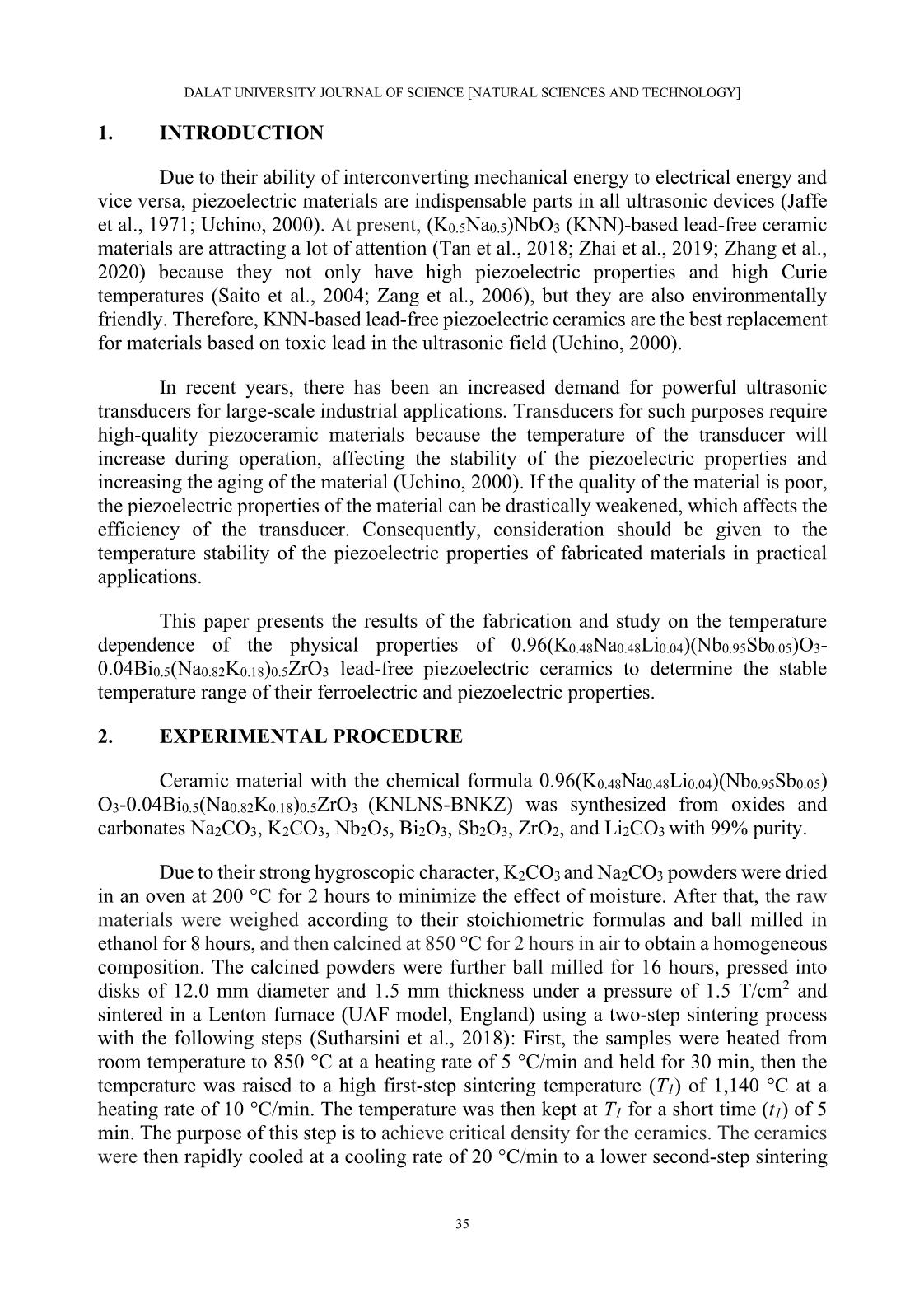
Trang 2
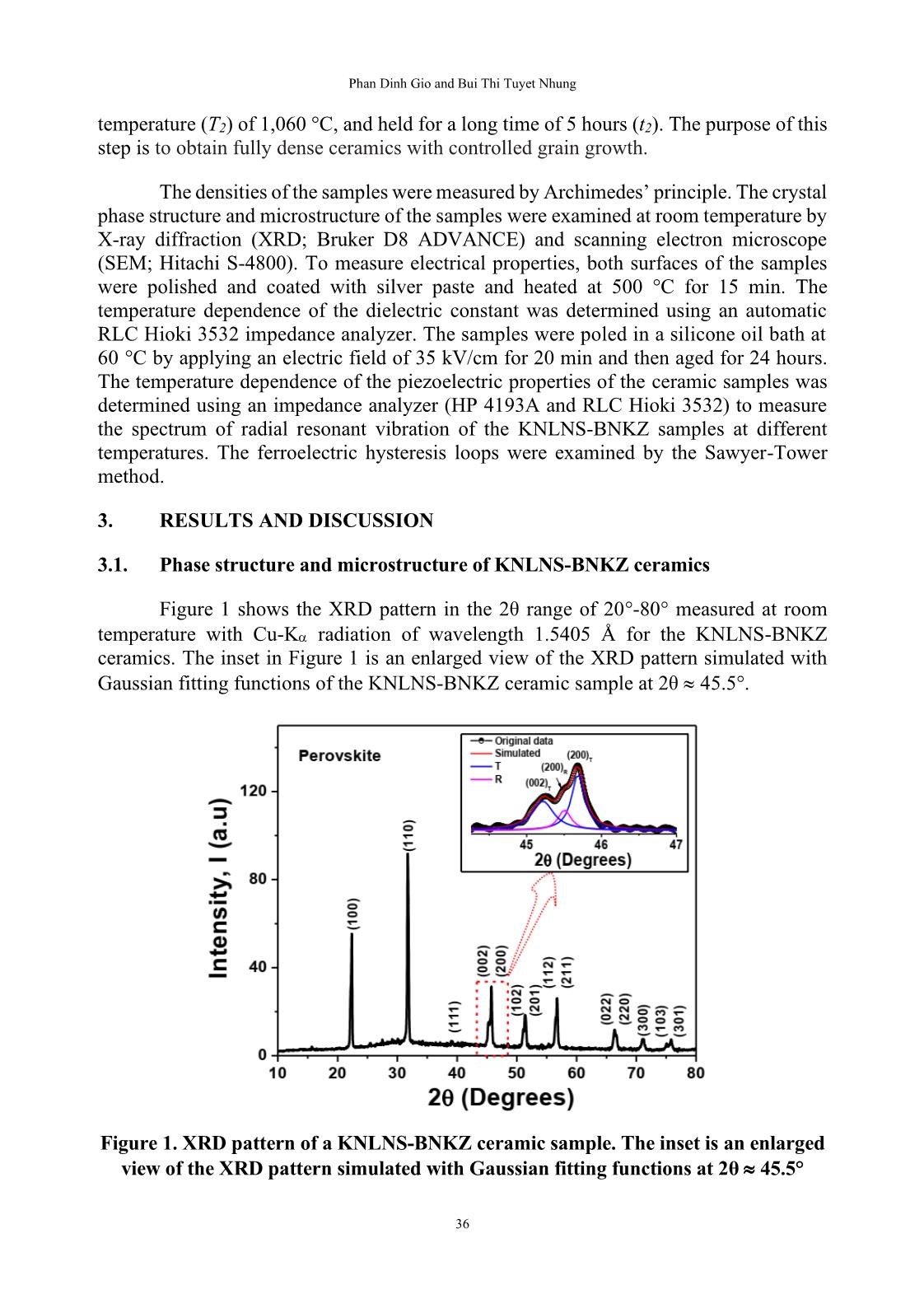
Trang 3
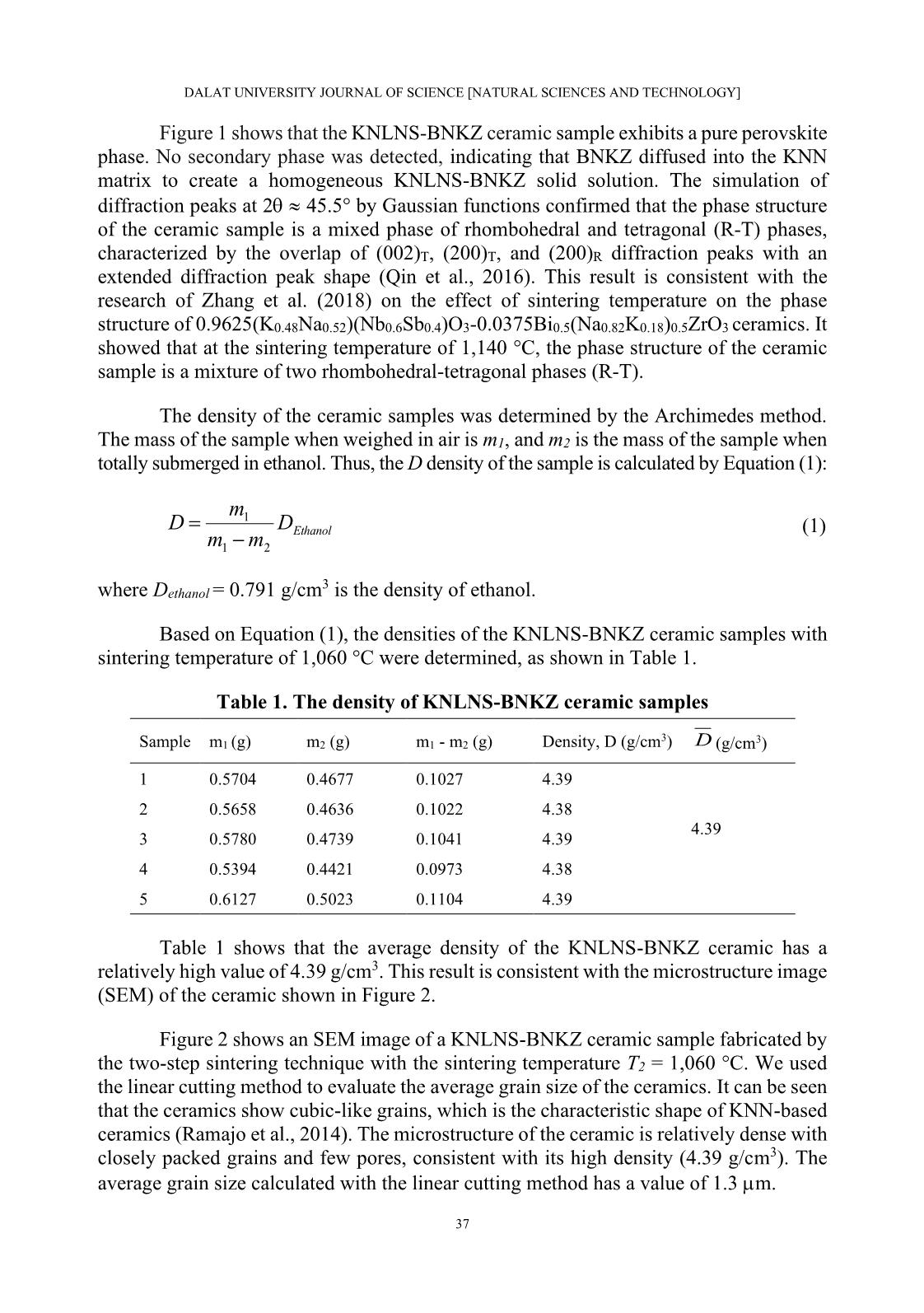
Trang 4
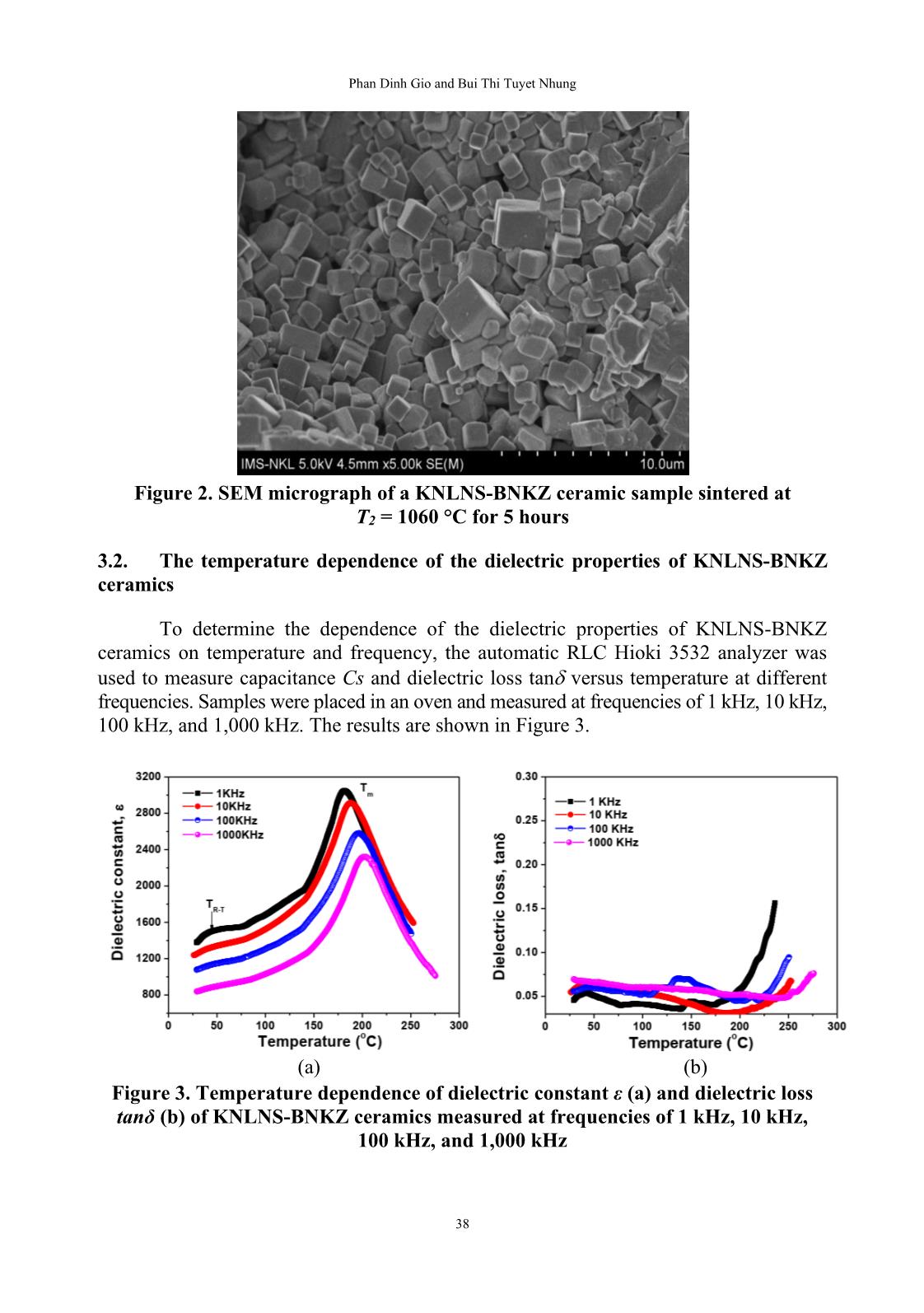
Trang 5
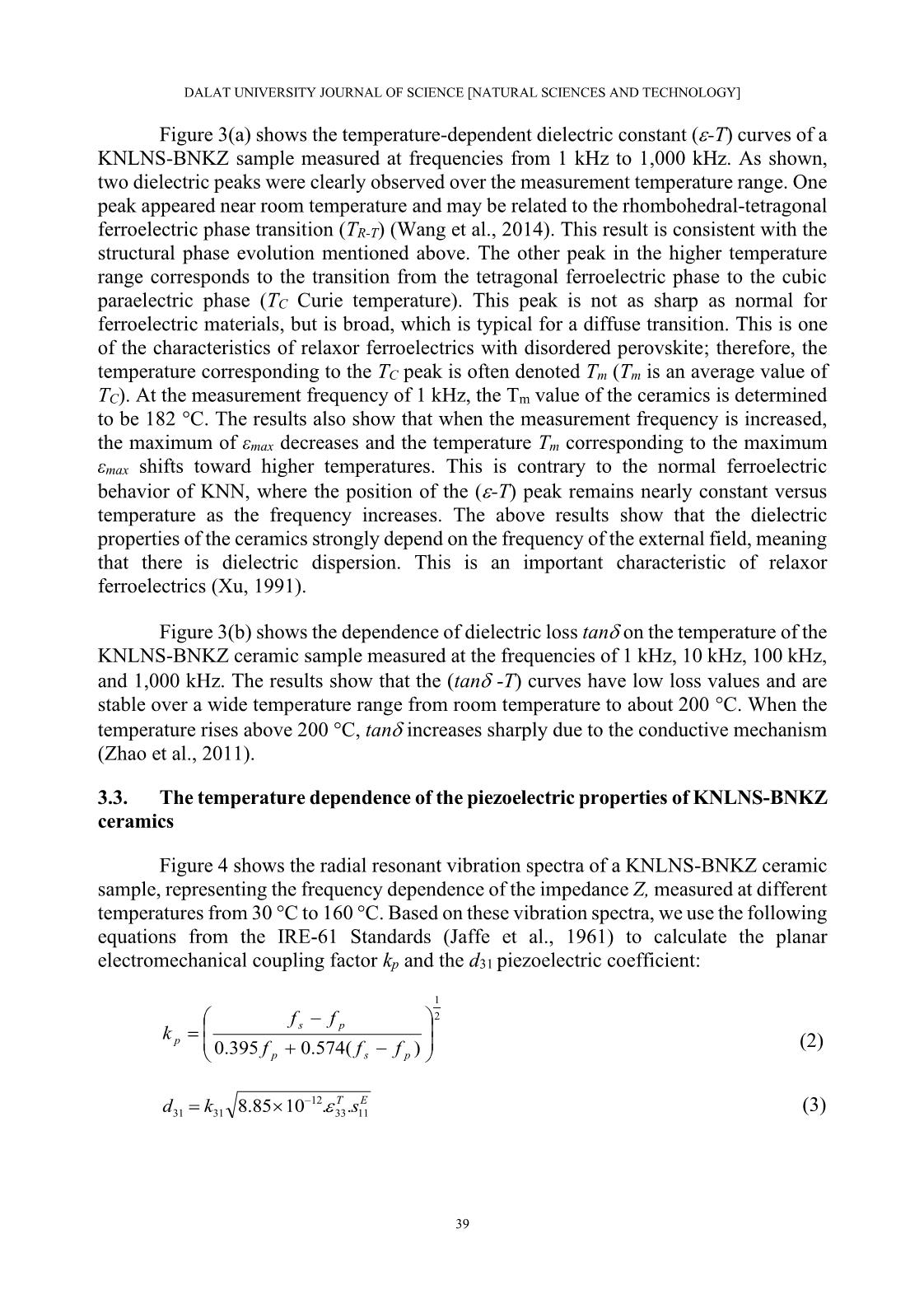
Trang 6
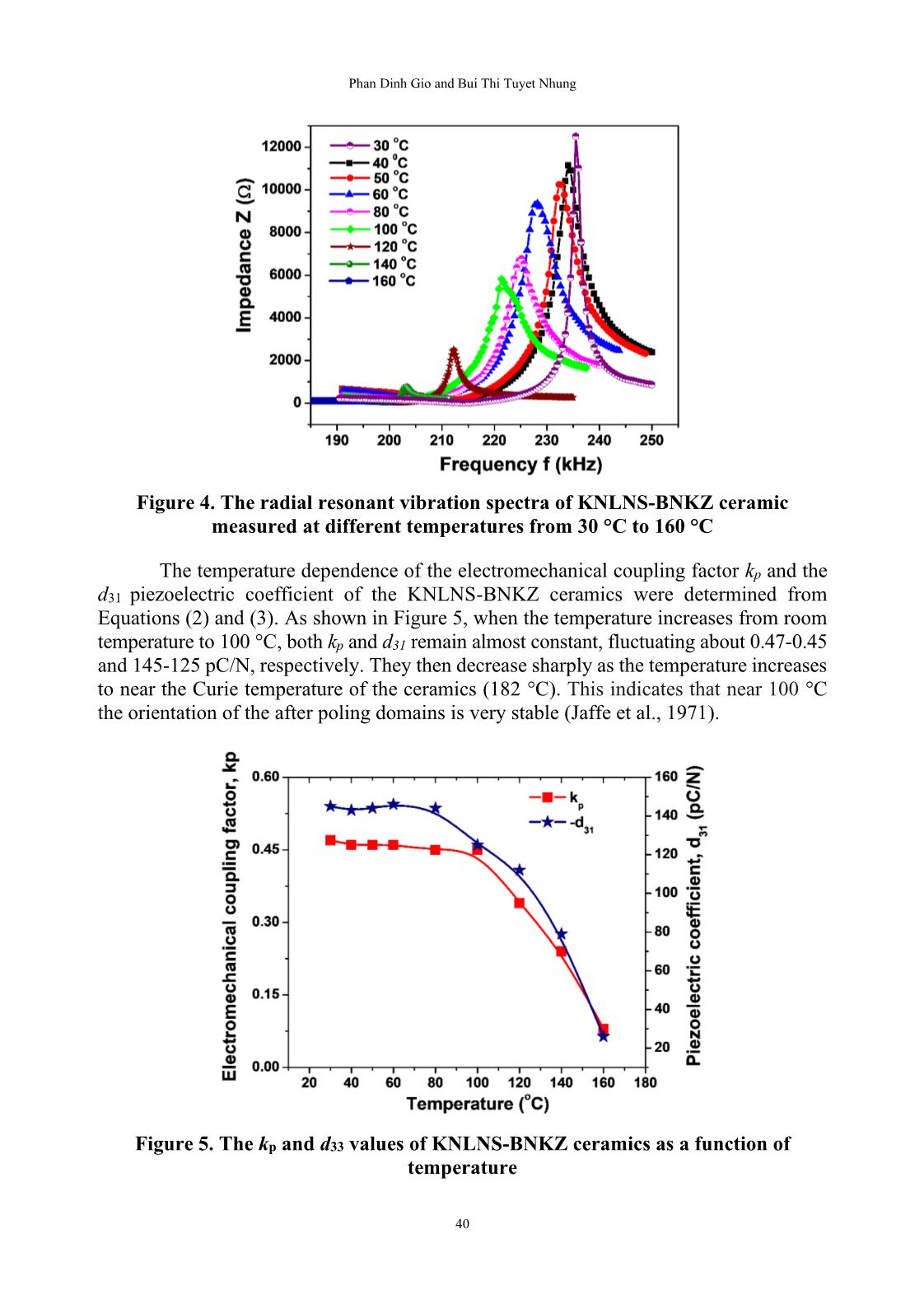
Trang 7
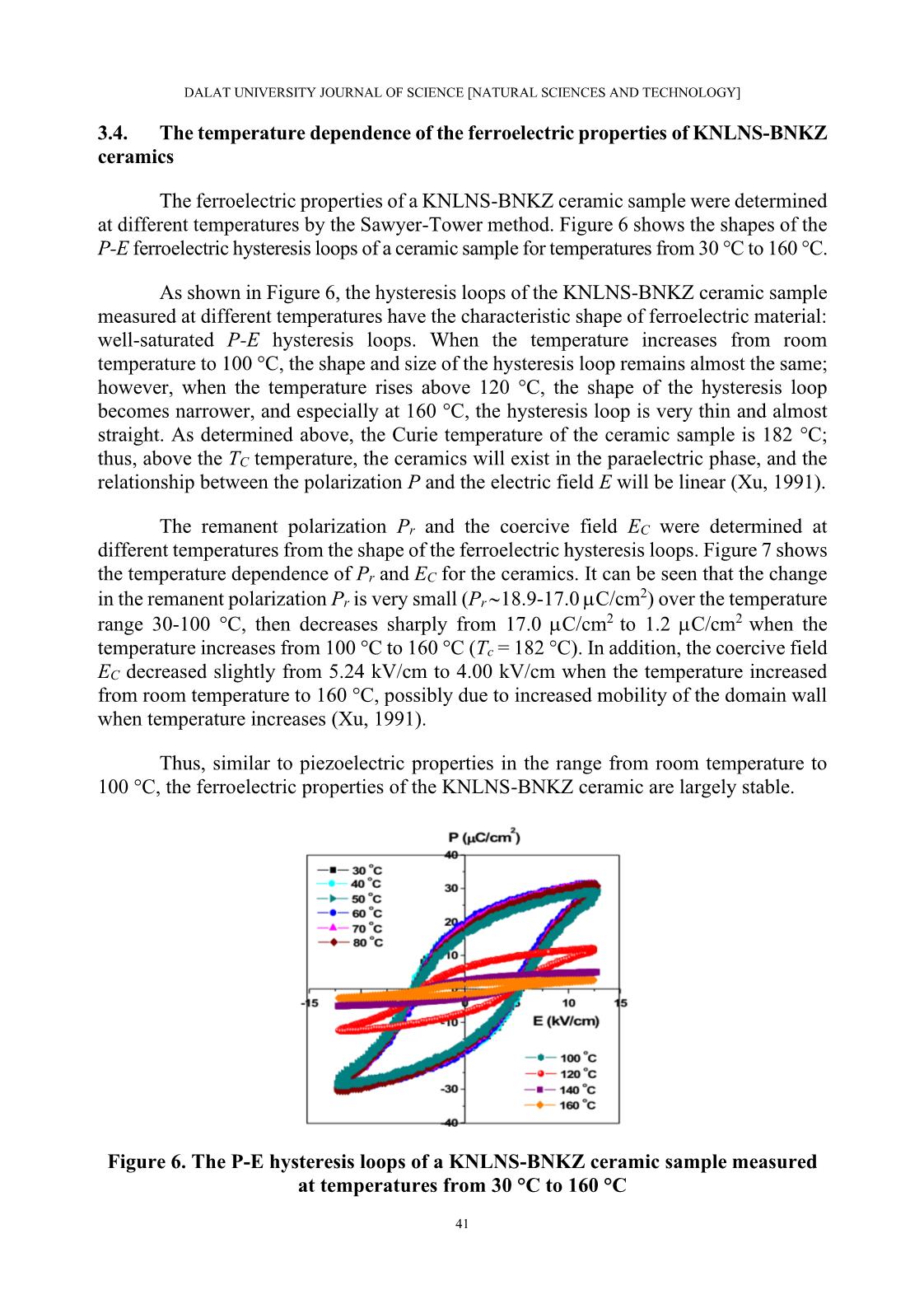
Trang 8
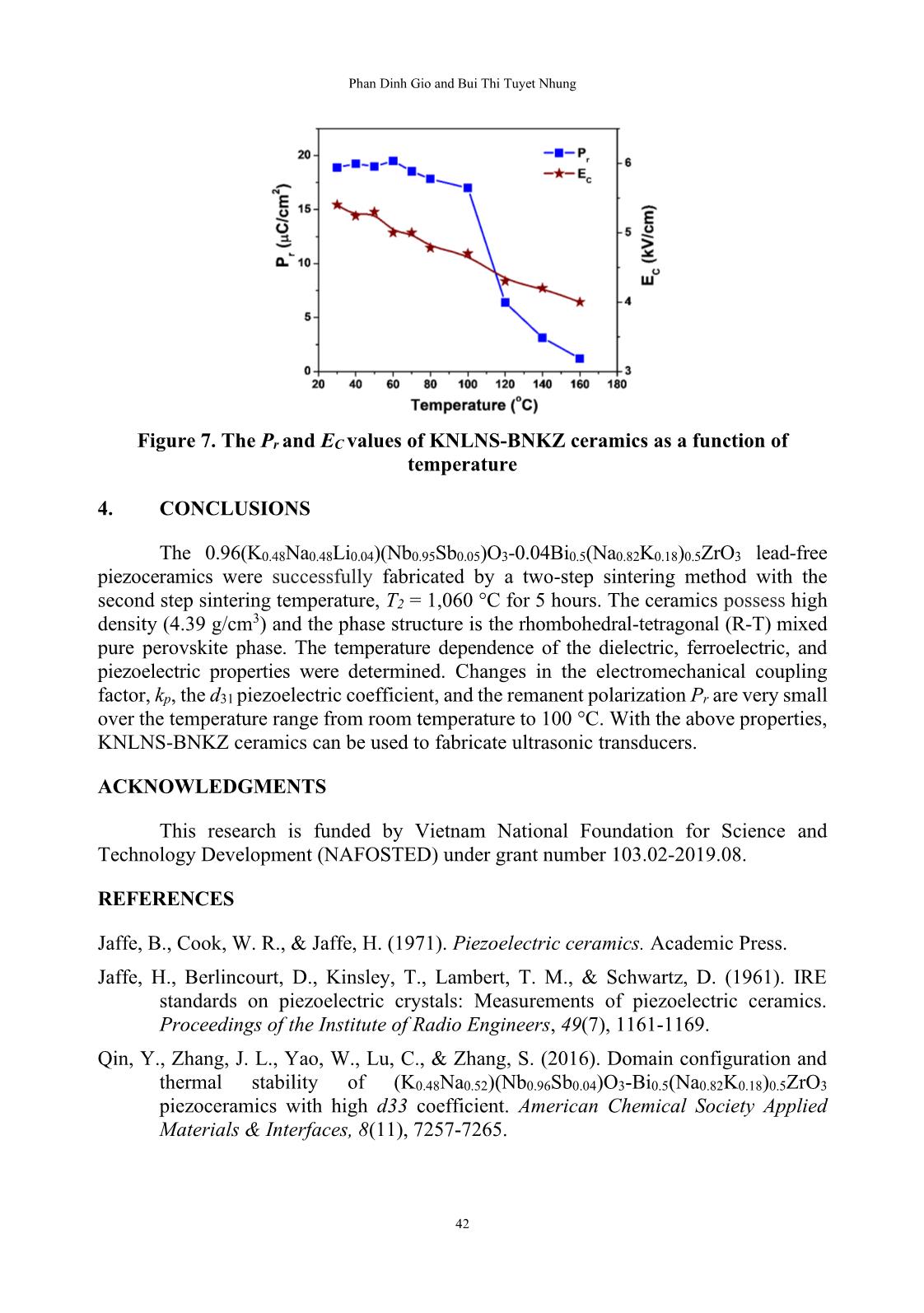
Trang 9
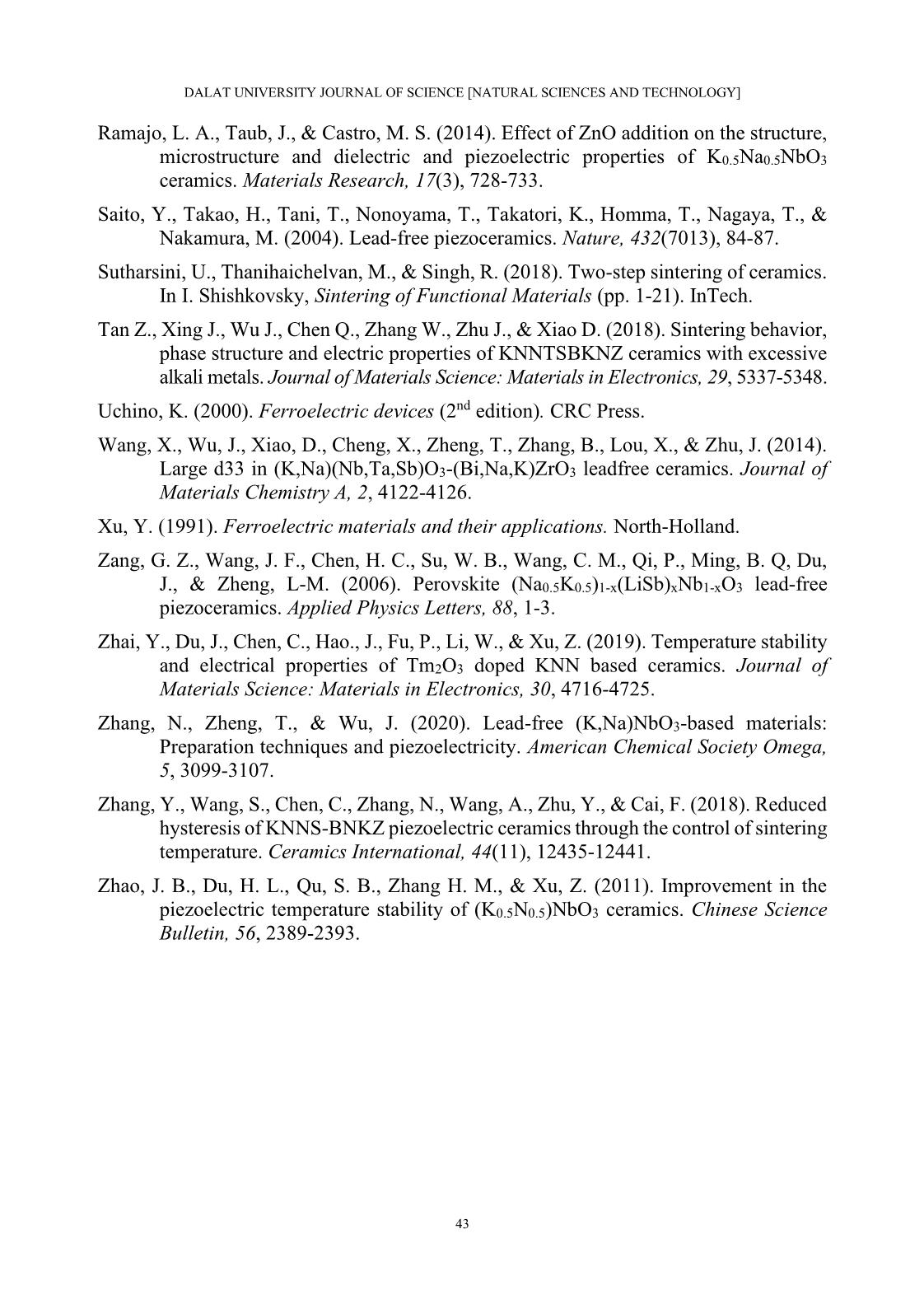
Trang 10
Tóm tắt nội dung tài liệu: Effect of temperature on dielectric, ferroelectric, and piezoelectric properties of knlns - bnkz ceramics
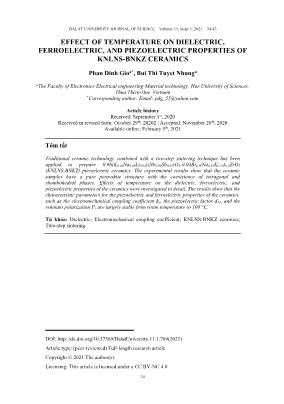
re measured by Archimedes’ principle. The crystal phase structure and microstructure of the samples were examined at room temperature by X-ray diffraction (XRD; Bruker D8 ADVANCE) and scanning electron microscope (SEM; Hitachi S-4800). To measure electrical properties, both surfaces of the samples were polished and coated with silver paste and heated at 500 °C for 15 min. The temperature dependence of the dielectric constant was determined using an automatic RLC Hioki 3532 impedance analyzer. The samples were poled in a silicone oil bath at 60 °C by applying an electric field of 35 kV/cm for 20 min and then aged for 24 hours. The temperature dependence of the piezoelectric properties of the ceramic samples was determined using an impedance analyzer (HP 4193A and RLC Hioki 3532) to measure the spectrum of radial resonant vibration of the KNLNS-BNKZ samples at different temperatures. The ferroelectric hysteresis loops were examined by the Sawyer-Tower method. 3. RESULTS AND DISCUSSION 3.1. Phase structure and microstructure of KNLNS-BNKZ ceramics Figure 1 shows the XRD pattern in the 2θ range of 20°-80° measured at room temperature with Cu-K radiation of wavelength 1.5405 Å for the KNLNS-BNKZ ceramics. The inset in Figure 1 is an enlarged view of the XRD pattern simulated with Gaussian fitting functions of the KNLNS-BNKZ ceramic sample at 2θ 45.5°. Figure 1. XRD pattern of a KNLNS-BNKZ ceramic sample. The inset is an enlarged view of the XRD pattern simulated with Gaussian fitting functions at 2θ 45.5° DALAT UNIVERSITY JOURNAL OF SCIENCE [NATURAL SCIENCES AND TECHNOLOGY] 37 Figure 1 shows that the KNLNS-BNKZ ceramic sample exhibits a pure perovskite phase. No secondary phase was detected, indicating that BNKZ diffused into the KNN matrix to create a homogeneous KNLNS-BNKZ solid solution. The simulation of diffraction peaks at 2 45.5° by Gaussian functions confirmed that the phase structure of the ceramic sample is a mixed phase of rhombohedral and tetragonal (R-T) phases, characterized by the overlap of (002)T, (200)T, and (200)R diffraction peaks with an extended diffraction peak shape (Qin et al., 2016). This result is consistent with the research of Zhang et al. (2018) on the effect of sintering temperature on the phase structure of 0.9625(K0.48Na0.52)(Nb0.6Sb0.4)O3-0.0375Bi0.5(Na0.82K0.18)0.5ZrO3 ceramics. It showed that at the sintering temperature of 1,140 °C, the phase structure of the ceramic sample is a mixture of two rhombohedral-tetragonal phases (R-T). The density of the ceramic samples was determined by the Archimedes method. The mass of the sample when weighed in air is m1, and m2 is the mass of the sample when totally submerged in ethanol. Thus, the D density of the sample is calculated by Equation (1): EthanolD mm m D 21 1 − = where Dethanol = 0.791 g/cm 3 is the density of ethanol. Based on Equation (1), the densities of the KNLNS-BNKZ ceramic samples with sintering temperature of 1,060 °C were determined, as shown in Table 1. Table 1. The density of KNLNS-BNKZ ceramic samples Sample m1 (g) m2 (g) m1 - m2 (g) Density, D (g/cm3) D (g/cm3) 1 0.5704 0.4677 0.1027 4.39 4.39 2 0.5658 0.4636 0.1022 4.38 3 0.5780 0.4739 0.1041 4.39 4 0.5394 0.4421 0.0973 4.38 5 0.6127 0.5023 0.1104 4.39 Table 1 shows that the average density of the KNLNS-BNKZ ceramic has a relatively high value of 4.39 g/cm3. This result is consistent with the microstructure image (SEM) of the ceramic shown in Figure 2. Figure 2 shows an SEM image of a KNLNS-BNKZ ceramic sample fabricated by the two-step sintering technique with the sintering temperature T2 = 1,060 °C. We used the linear cutting method to evaluate the average grain size of the ceramics. It can be seen that the ceramics show cubic-like grains, which is the characteristic shape of KNN-based ceramics (Ramajo et al., 2014). The microstructure of the ceramic is relatively dense with closely packed grains and few pores, consistent with its high density (4.39 g/cm3). The average grain size calculated with the linear cutting method has a value of 1.3 m. (1) Phan Dinh Gio and Bui Thi Tuyet Nhung 38 Figure 2. SEM micrograph of a KNLNS-BNKZ ceramic sample sintered at T2 = 1060 °C for 5 hours 3.2. The temperature dependence of the dielectric properties of KNLNS-BNKZ ceramics To determine the dependence of the dielectric properties of KNLNS-BNKZ ceramics on temperature and frequency, the automatic RLC Hioki 3532 analyzer was used to measure capacitance Cs and dielectric loss tan versus temperature at different frequencies. Samples were placed in an oven and measured at frequencies of 1 kHz, 10 kHz, 100 kHz, and 1,000 kHz. The results are shown in Figure 3. Figure 3. Temperature dependence of dielectric constant ε (a) and dielectric loss tanδ (b) of KNLNS-BNKZ ceramics measured at frequencies of 1 kHz, 10 kHz, 100 kHz, and 1,000 kHz (a) (b) DALAT UNIVERSITY JOURNAL OF SCIENCE [NATURAL SCIENCES AND TECHNOLOGY] 39 Figure 3(a) shows the temperature-dependent dielectric constant (-T) curves of a KNLNS-BNKZ sample measured at frequencies from 1 kHz to 1,000 kHz. As shown, two dielectric peaks were clearly observed over the measurement temperature range. One peak appeared near room temperature and may be related to the rhombohedral-tetragonal ferroelectric phase transition (TR-T) (Wang et al., 2014). This result is consistent with the structural phase evolution mentioned above. The other peak in the higher temperature range corresponds to the transition from the tetragonal ferroelectric phase to the cubic paraelectric phase (TC Curie temperature). This peak is not as sharp as normal for ferroelectric materials, but is broad, which is typical for a diffuse transition. This is one of the characteristics of relaxor ferroelectrics with disordered perovskite; therefore, the temperature corresponding to the TC peak is often denoted Tm (Tm is an average value of TC). At the measurement frequency of 1 kHz, the Tm value of the ceramics is determined to be 182 °C. The results also show that when the measurement frequency is increased, the maximum of εmax decreases and the temperature Tm corresponding to the maximum εmax shifts toward higher temperatures. This is contrary to the normal ferroelectric behavior of KNN, where the position of the (-T) peak remains nearly constant versus temperature as the frequency increases. The above results show that the dielectric properties of the ceramics strongly depend on the frequency of the external field, meaning that there is dielectric dispersion. This is an important characteristic of relaxor ferroelectrics (Xu, 1991). Figure 3(b) shows the dependence of dielectric loss tan on the temperature of the KNLNS-BNKZ ceramic sample measured at the frequencies of 1 kHz, 10 kHz, 100 kHz, and 1,000 kHz. The results show that the (tan -T) curves have low loss values and are stable over a wide temperature range from room temperature to about 200 °C. When the temperature rises above 200 °C, tan increases sharply due to the conductive mechanism (Zhao et al., 2011). 3.3. The temperature dependence of the piezoelectric properties of KNLNS-BNKZ ceramics Figure 4 shows the radial resonant vibration spectra of a KNLNS-BNKZ ceramic sample, representing the frequency dependence of the impedance Z, measured at different temperatures from 30 °C to 160 °C. Based on these vibration spectra, we use the following equations from the IRE-61 Standards (Jaffe et al., 1961) to calculate the planar electromechanical coupling factor kp and the d31 piezoelectric coefficient: 2 1 )(574.0395.0 −+ − = psp ps p fff ff k ET skd 1133 12 3131 ..1085.8 − = (3) (2) Phan Dinh Gio and Bui Thi Tuyet Nhung 40 Figure 4. The radial resonant vibration spectra of KNLNS-BNKZ ceramic measured at different temperatures from 30 °C to 160 °C The temperature dependence of the electromechanical coupling factor kp and the d31 piezoelectric coefficient of the KNLNS-BNKZ ceramics were determined from Equations (2) and (3). As shown in Figure 5, when the temperature increases from room temperature to 100 °C, both kp and d31 remain almost constant, fluctuating about 0.47-0.45 and 145-125 pC/N, respectively. They then decrease sharply as the temperature increases to near the Curie temperature of the ceramics (182 °C). This indicates that near 100 °C the orientation of the after poling domains is very stable (Jaffe et al., 1971). Figure 5. The kp and d33 values of KNLNS-BNKZ ceramics as a function of temperature DALAT UNIVERSITY JOURNAL OF SCIENCE [NATURAL SCIENCES AND TECHNOLOGY] 41 3.4. The temperature dependence of the ferroelectric properties of KNLNS-BNKZ ceramics The ferroelectric properties of a KNLNS-BNKZ ceramic sample were determined at different temperatures by the Sawyer-Tower method. Figure 6 shows the shapes of the P-E ferroelectric hysteresis loops of a ceramic sample for temperatures from 30 °C to 160 °C. As shown in Figure 6, the hysteresis loops of the KNLNS-BNKZ ceramic sample measured at different temperatures have the characteristic shape of ferroelectric material: well-saturated P-E hysteresis loops. When the temperature increases from room temperature to 100 °C, the shape and size of the hysteresis loop remains almost the same; however, when the temperature rises above 120 °C, the shape of the hysteresis loop becomes narrower, and especially at 160 °C, the hysteresis loop is very thin and almost straight. As determined above, the Curie temperature of the ceramic sample is 182 °C; thus, above the TC temperature, the ceramics will exist in the paraelectric phase, and the relationship between the polarization P and the electric field E will be linear (Xu, 1991). The remanent polarization Pr and the coercive field EC were determined at different temperatures from the shape of the ferroelectric hysteresis loops. Figure 7 shows the temperature dependence of Pr and EC for the ceramics. It can be seen that the change in the remanent polarization Pr is very small (Pr 18.9-17.0 C/cm2) over the temperature range 30-100 °C, then decreases sharply from 17.0 C/cm2 to 1.2 C/cm2 when the temperature increases from 100 °C to 160 °C (Tc = 182 °C). In addition, the coercive field EC decreased slightly from 5.24 kV/cm to 4.00 kV/cm when the temperature increased from room temperature to 160 °C, possibly due to increased mobility of the domain wall when temperature increases (Xu, 1991). Thus, similar to piezoelectric properties in the range from room temperature to 100 °C, the ferroelectric properties of the KNLNS-BNKZ ceramic are largely stable. Figure 6. The P-E hysteresis loops of a KNLNS-BNKZ ceramic sample measured at temperatures from 30 °C to 160 °C Phan Dinh Gio and Bui Thi Tuyet Nhung 42 Figure 7. The Pr and EC values of KNLNS-BNKZ ceramics as a function of temperature 4. CONCLUSIONS The 0.96(K0.48Na0.48Li0.04)(Nb0.95Sb0.05)O3-0.04Bi0.5(Na0.82K0.18)0.5ZrO3 lead-free piezoceramics were successfully fabricated by a two-step sintering method with the second step sintering temperature, T2 = 1,060 °C for 5 hours. The ceramics possess high density (4.39 g/cm3) and the phase structure is the rhombohedral-tetragonal (R-T) mixed pure perovskite phase. The temperature dependence of the dielectric, ferroelectric, and piezoelectric properties were determined. Changes in the electromechanical coupling factor, kp, the d31 piezoelectric coefficient, and the remanent polarization Pr are very small over the temperature range from room temperature to 100 °C. With the above properties, KNLNS-BNKZ ceramics can be used to fabricate ultrasonic transducers. ACKNOWLEDGMENTS This research is funded by Vietnam National Foundation for Science and Technology Development (NAFOSTED) under grant number 103.02-2019.08. REFERENCES Jaffe, B., Cook, W. R., & Jaffe, H. (1971). Piezoelectric ceramics. Academic Press. Jaffe, H., Berlincourt, D., Kinsley, T., Lambert, T. M., & Schwartz, D. (1961). IRE standards on piezoelectric crystals: Measurements of piezoelectric ceramics. Proceedings of the Institute of Radio Engineers, 49(7), 1161-1169. Qin, Y., Zhang, J. L., Yao, W., Lu, C., & Zhang, S. (2016). Domain configuration and thermal stability of (K0.48Na0.52)(Nb0.96Sb0.04)O3-Bi0.5(Na0.82K0.18)0.5ZrO3 piezoceramics with high d33 coefficient. American Chemical Society Applied Materials & Interfaces, 8(11), 7257-7265. DALAT UNIVERSITY JOURNAL OF SCIENCE [NATURAL SCIENCES AND TECHNOLOGY] 43 Ramajo, L. A., Taub, J., & Castro, M. S. (2014). Effect of ZnO addition on the structure, microstructure and dielectric and piezoelectric properties of K0.5Na0.5NbO3 ceramics. Materials Research, 17(3), 728-733. Saito, Y., Takao, H., Tani, T., Nonoyama, T., Takatori, K., Homma, T., Nagaya, T., & Nakamura, M. (2004). Lead-free piezoceramics. Nature, 432(7013), 84-87. Sutharsini, U., Thanihaichelvan, M., & Singh, R. (2018). Two-step sintering of ceramics. In I. Shishkovsky, Sintering of Functional Materials (pp. 1-21). InTech. Tan Z., Xing J., Wu J., Chen Q., Zhang W., Zhu J., & Xiao D. (2018). Sintering behavior, phase structure and electric properties of KNNTSBKNZ ceramics with excessive alkali metals. Journal of Materials Science: Materials in Electronics, 29, 5337-5348. Uchino, K. (2000). Ferroelectric devices (2nd edition). CRC Press. Wang, X., Wu, J., Xiao, D., Cheng, X., Zheng, T., Zhang, B., Lou, X., & Zhu, J. (2014). Large d33 in (K,Na)(Nb,Ta,Sb)O3-(Bi,Na,K)ZrO3 leadfree ceramics. Journal of Materials Chemistry A, 2, 4122-4126. Xu, Y. (1991). Ferroelectric materials and their applications. North-Holland. Zang, G. Z., Wang, J. F., Chen, H. C., Su, W. B., Wang, C. M., Qi, P., Ming, B. Q, Du, J., & Zheng, L-M. (2006). Perovskite (Na0.5K0.5)1-x(LiSb)xNb1-xO3 lead-free piezoceramics. Applied Physics Letters, 88, 1-3. Zhai, Y., Du, J., Chen, C., Hao., J., Fu, P., Li, W., & Xu, Z. (2019). Temperature stability and electrical properties of Tm2O3 doped KNN based ceramics. Journal of Materials Science: Materials in Electronics, 30, 4716-4725. Zhang, N., Zheng, T., & Wu, J. (2020). Lead-free (K,Na)NbO3-based materials: Preparation techniques and piezoelectricity. American Chemical Society Omega, 5, 3099-3107. Zhang, Y., Wang, S., Chen, C., Zhang, N., Wang, A., Zhu, Y., & Cai, F. (2018). Reduced hysteresis of KNNS-BNKZ piezoelectric ceramics through the control of sintering temperature. Ceramics International, 44(11), 12435-12441. Zhao, J. B., Du, H. L., Qu, S. B., Zhang H. M., & Xu, Z. (2011). Improvement in the piezoelectric temperature stability of (K0.5N0.5)NbO3 ceramics. Chinese Science Bulletin, 56, 2389-2393.
File đính kèm:
effect_of_temperature_on_dielectric_ferroelectric_and_piezoe.pdf