Development of a tunable ultrashort dye laser system by using molecular photonic processes and a nanosecond pumping laser
The paper presents the physical processes and operational characteristics of an original subpicosecond dye laser system which is designed and successfully developed at our laboratory
basing on the combination use of three photonic processes of dye molecules: 1) Fast spectrotemporal evolutions in the broadband dye laser emissions; 2) Generation of a chain of ultrashort
pulses (spikes) from low-Q micro-cavity dye lasers; and 3) Non-linear resonant interaction
between ultrashort laser pulses and highly-saturated dye media. Such a laser system provides a
stable generation of single 500 fs pulses with a peak power of 300 MW at 606 nm. Particularly,
the whole sub-picosecond laser system is pumped by a single nanosecond laser (Q-switched
Nd:YAG laser). The spectral and time processes involved in these pulse-shortening methods are
analyzed with a rate-equation model extended to wavelengths. A white light continuum
generation was obtained by focusing the 500 fs pulses into 2 cm water cell. As a result, it
enables us to produce widely tunable ultrashort laser pulses with a spectral selection and
amplification of the supercontinuum spectrum.
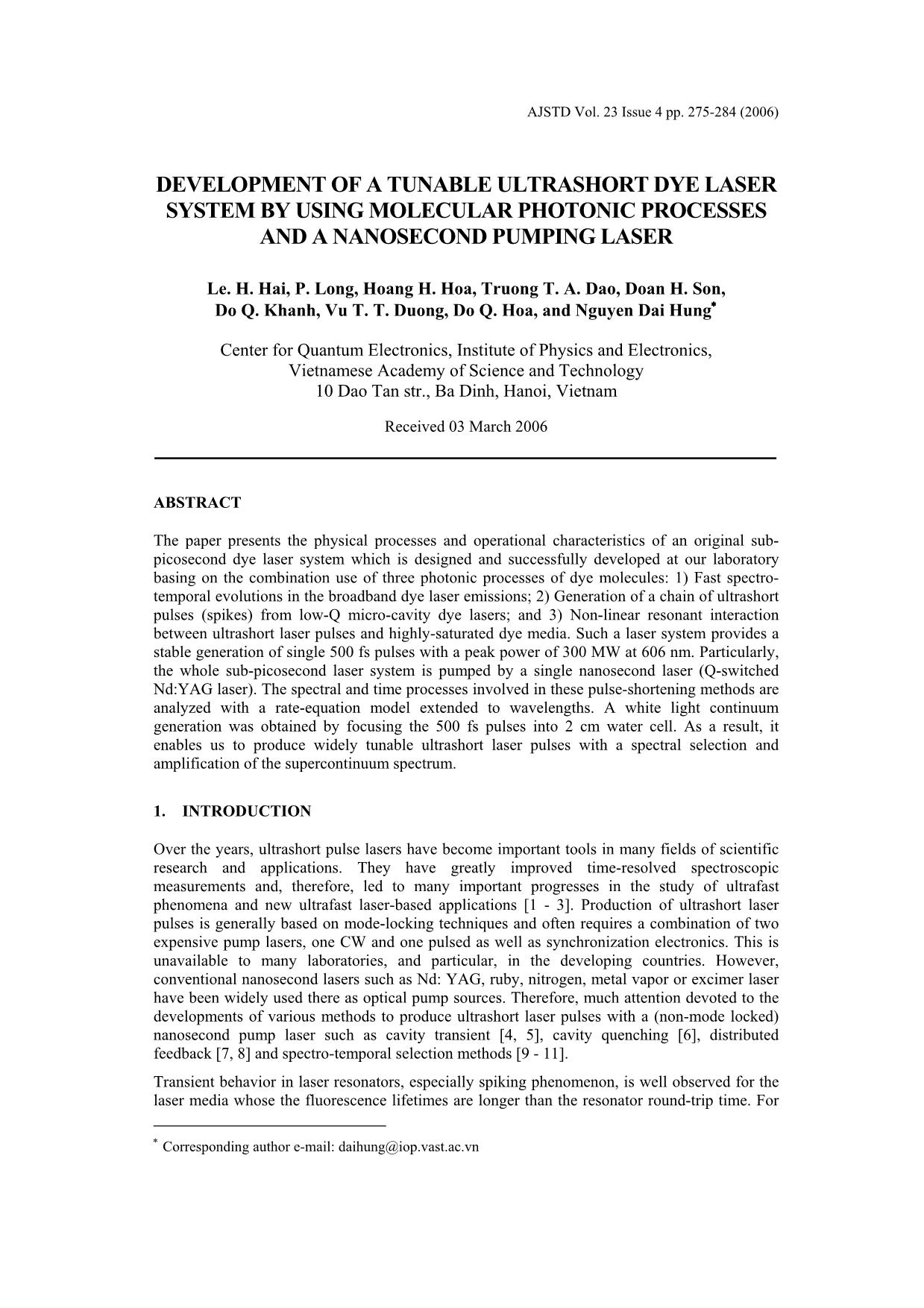
Trang 1
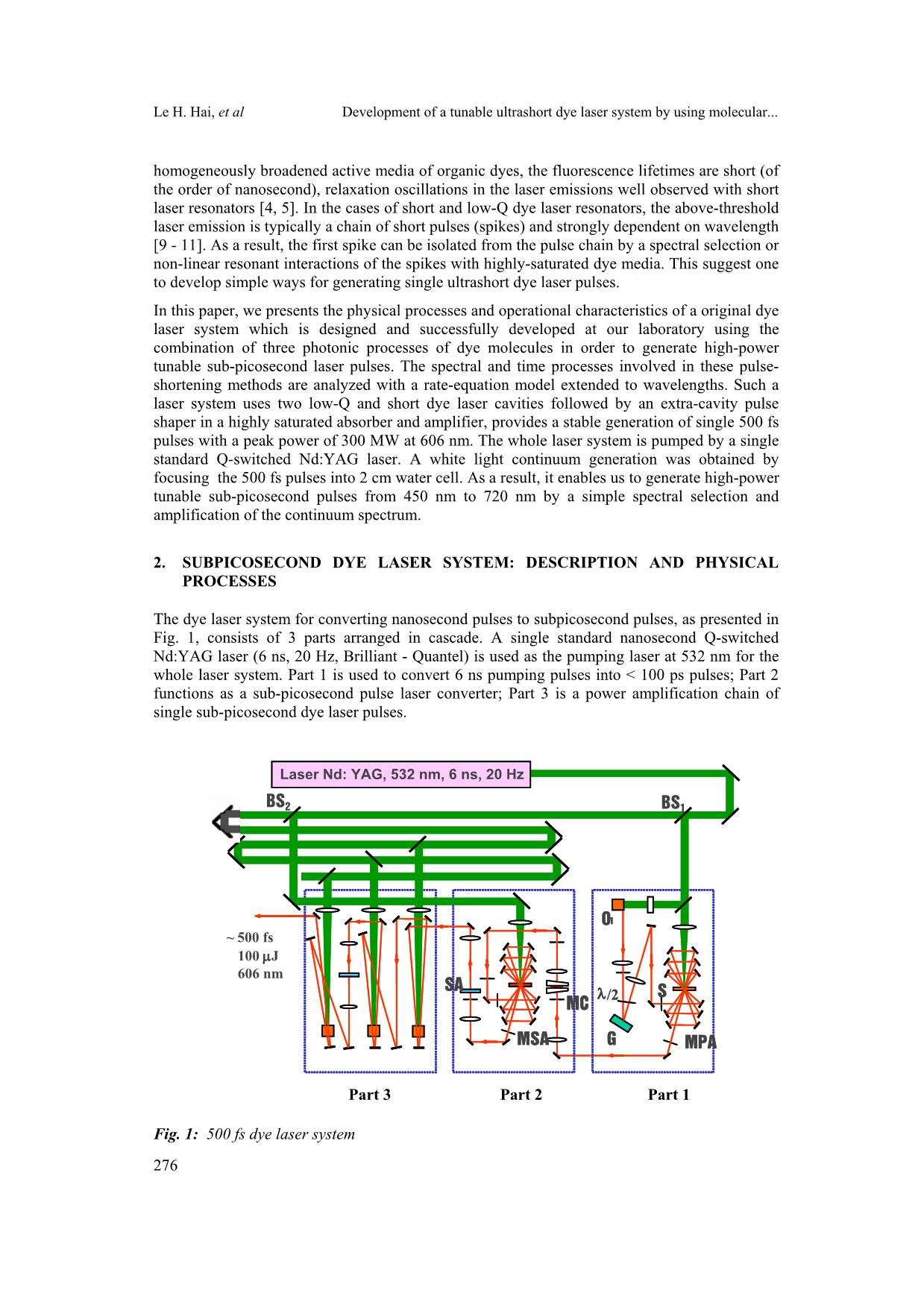
Trang 2
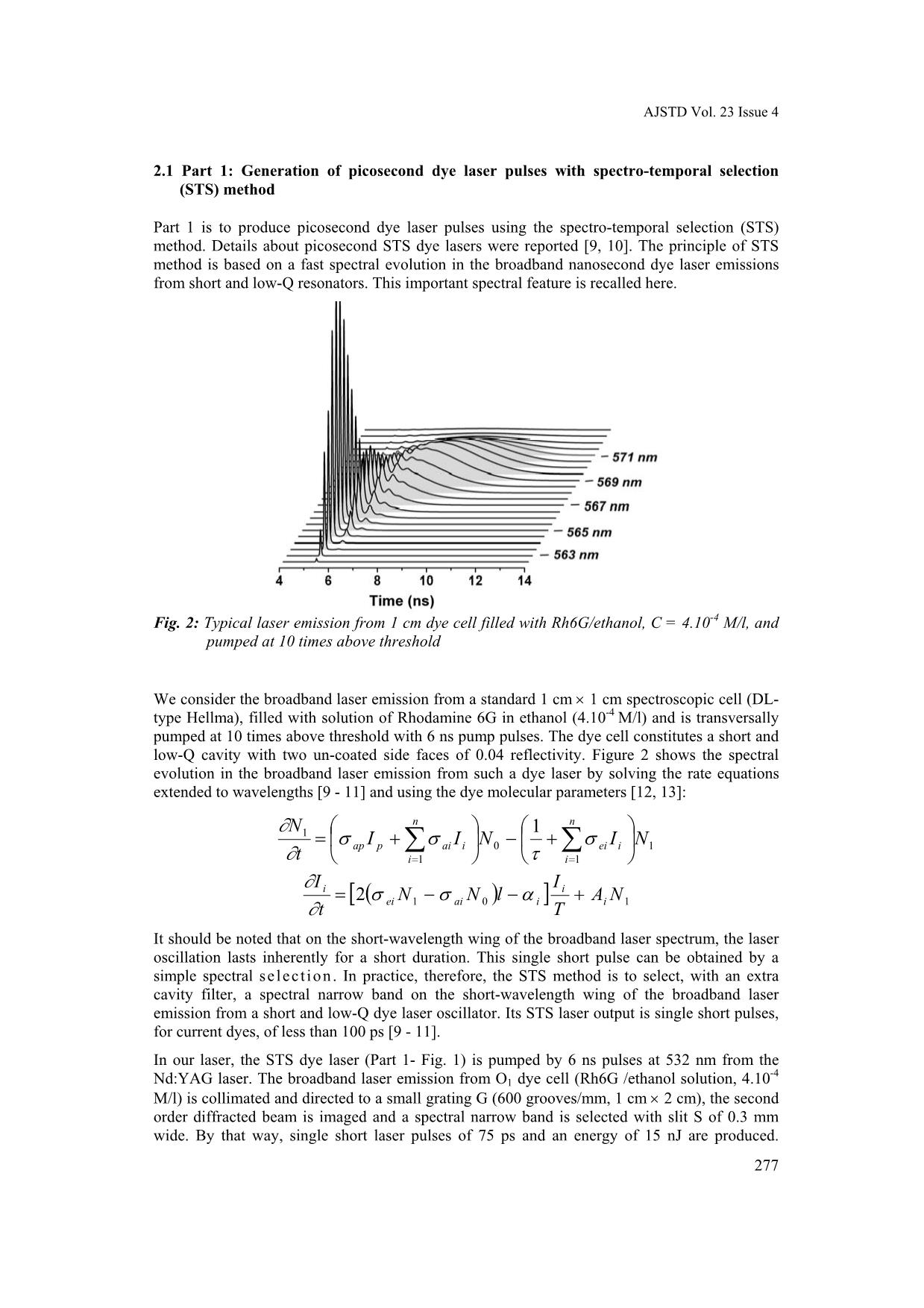
Trang 3
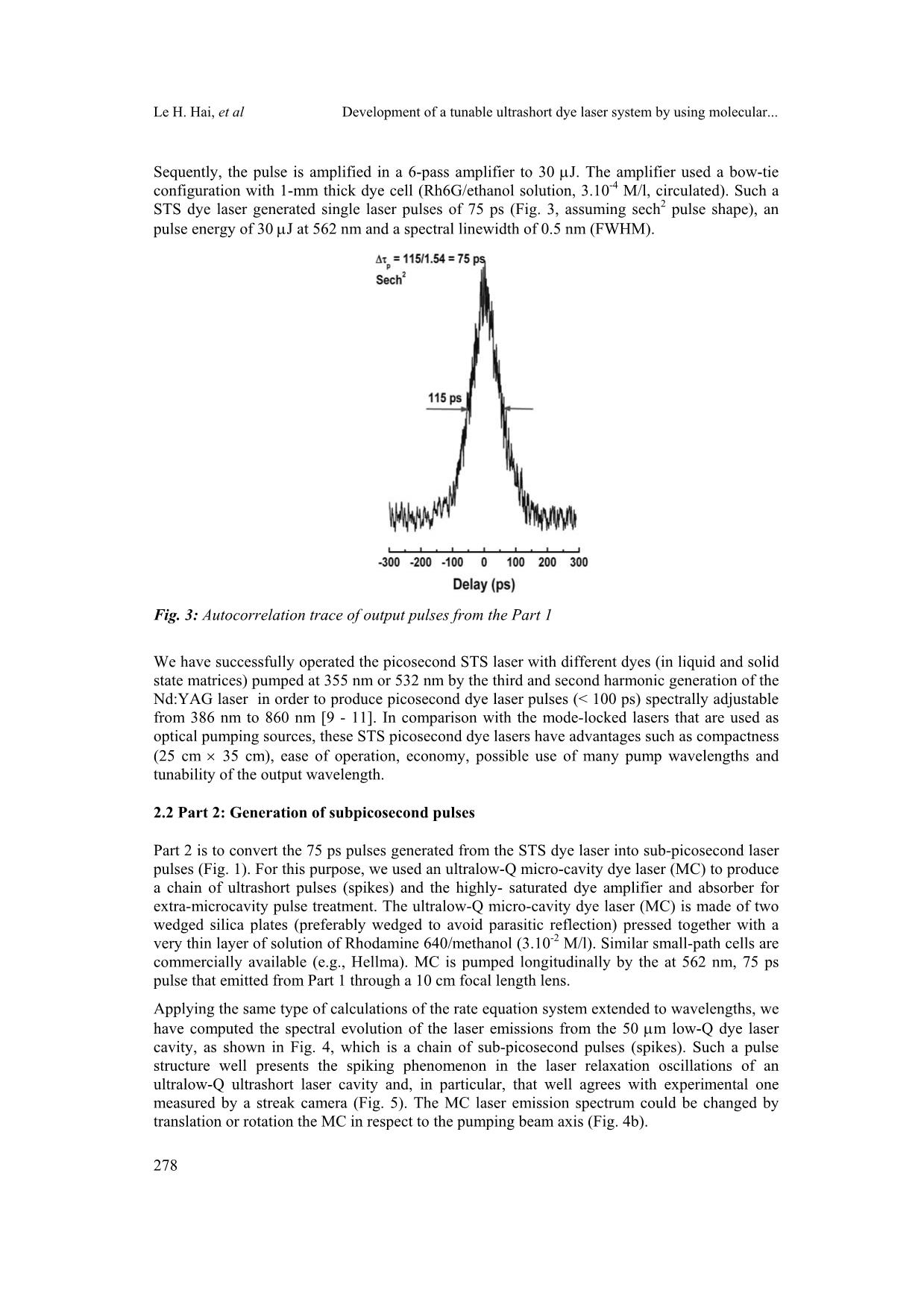
Trang 4
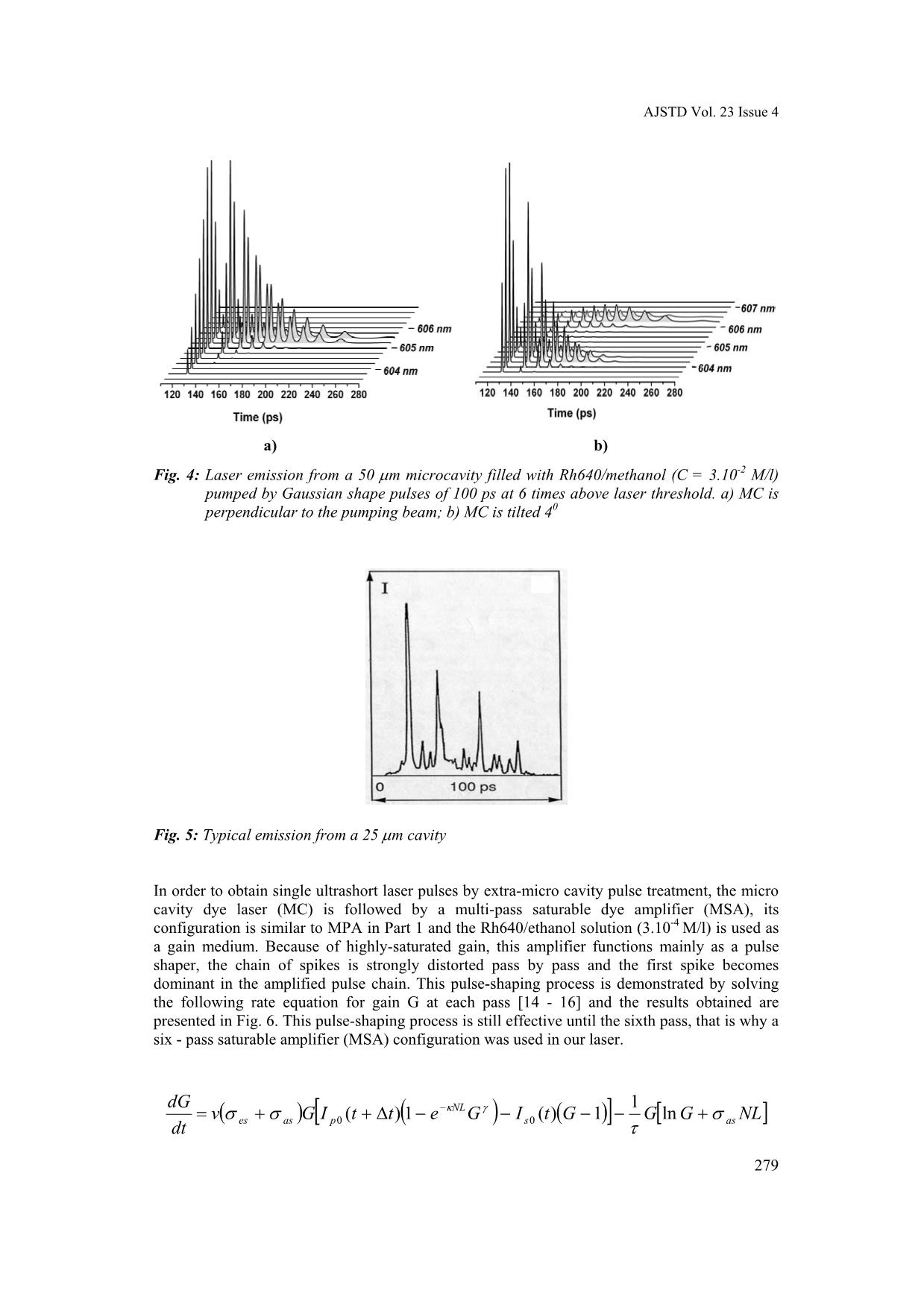
Trang 5
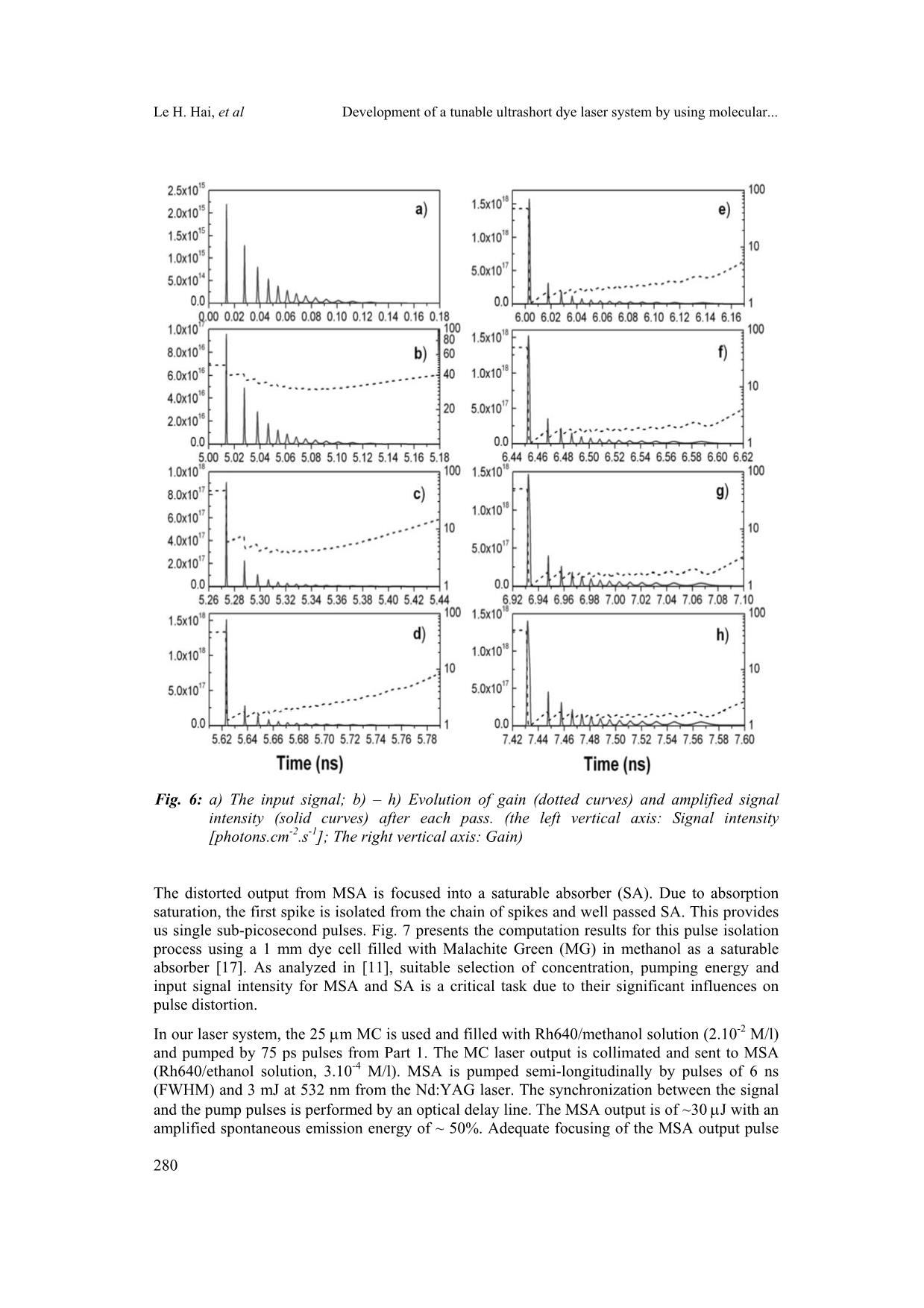
Trang 6
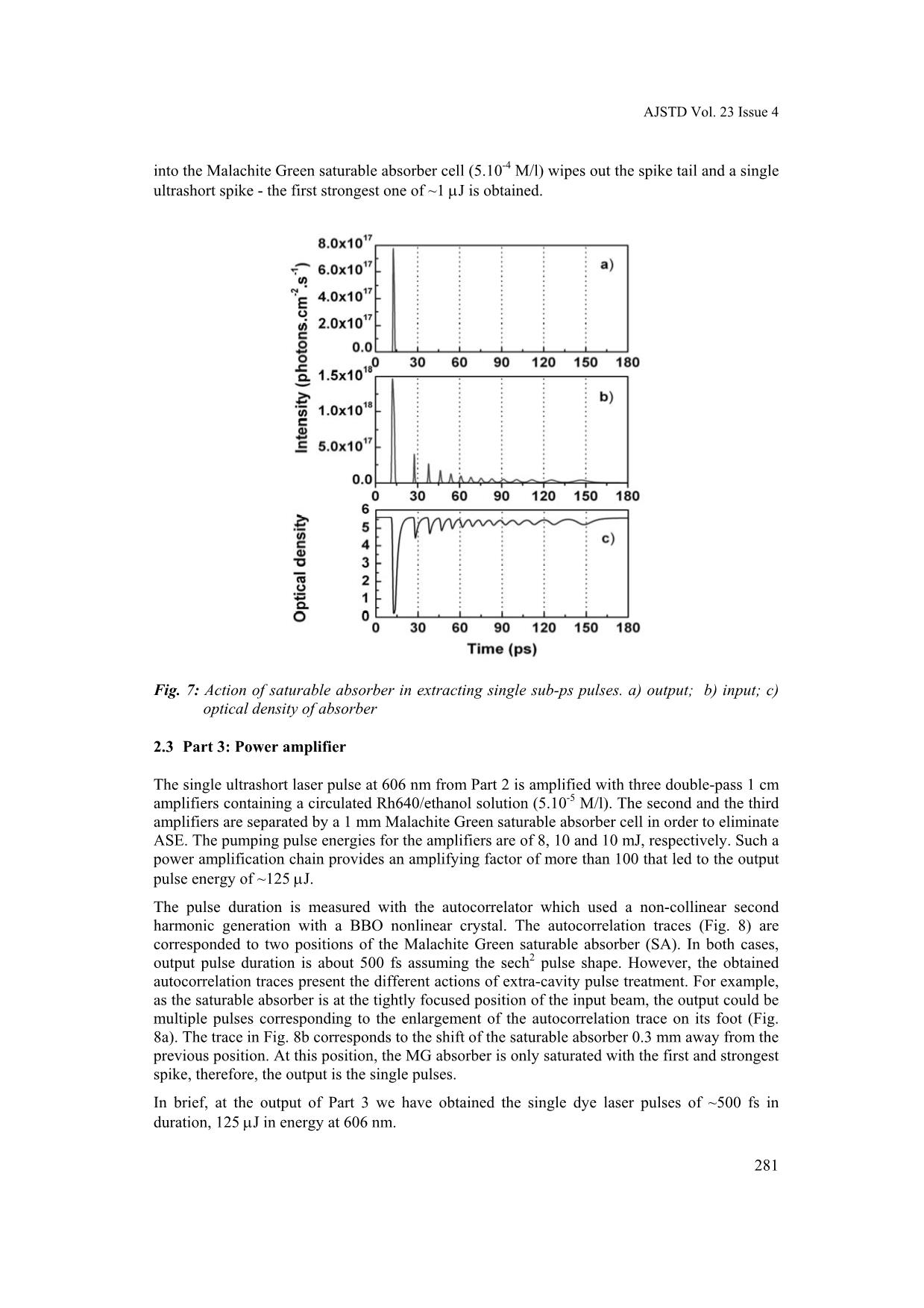
Trang 7
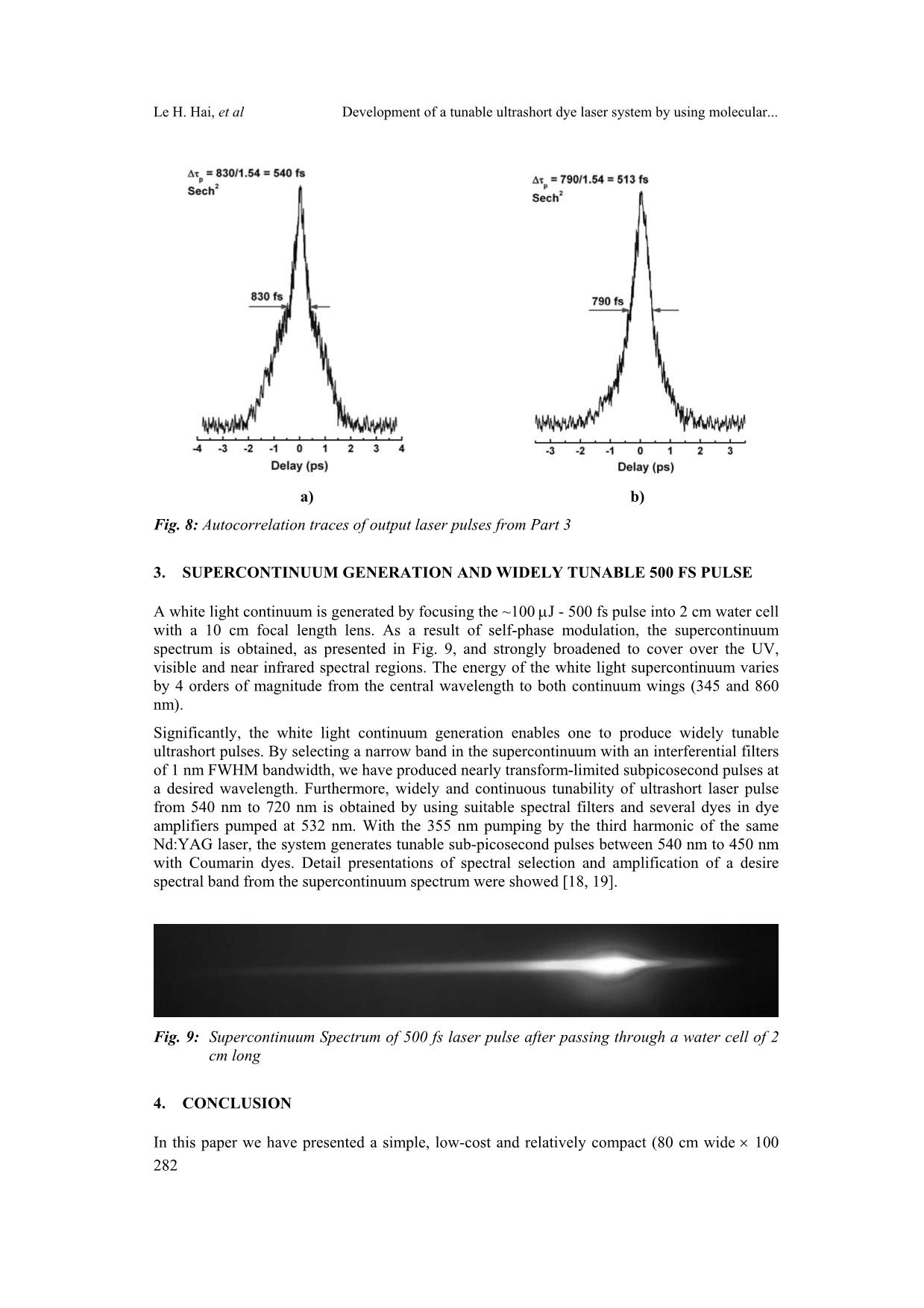
Trang 8
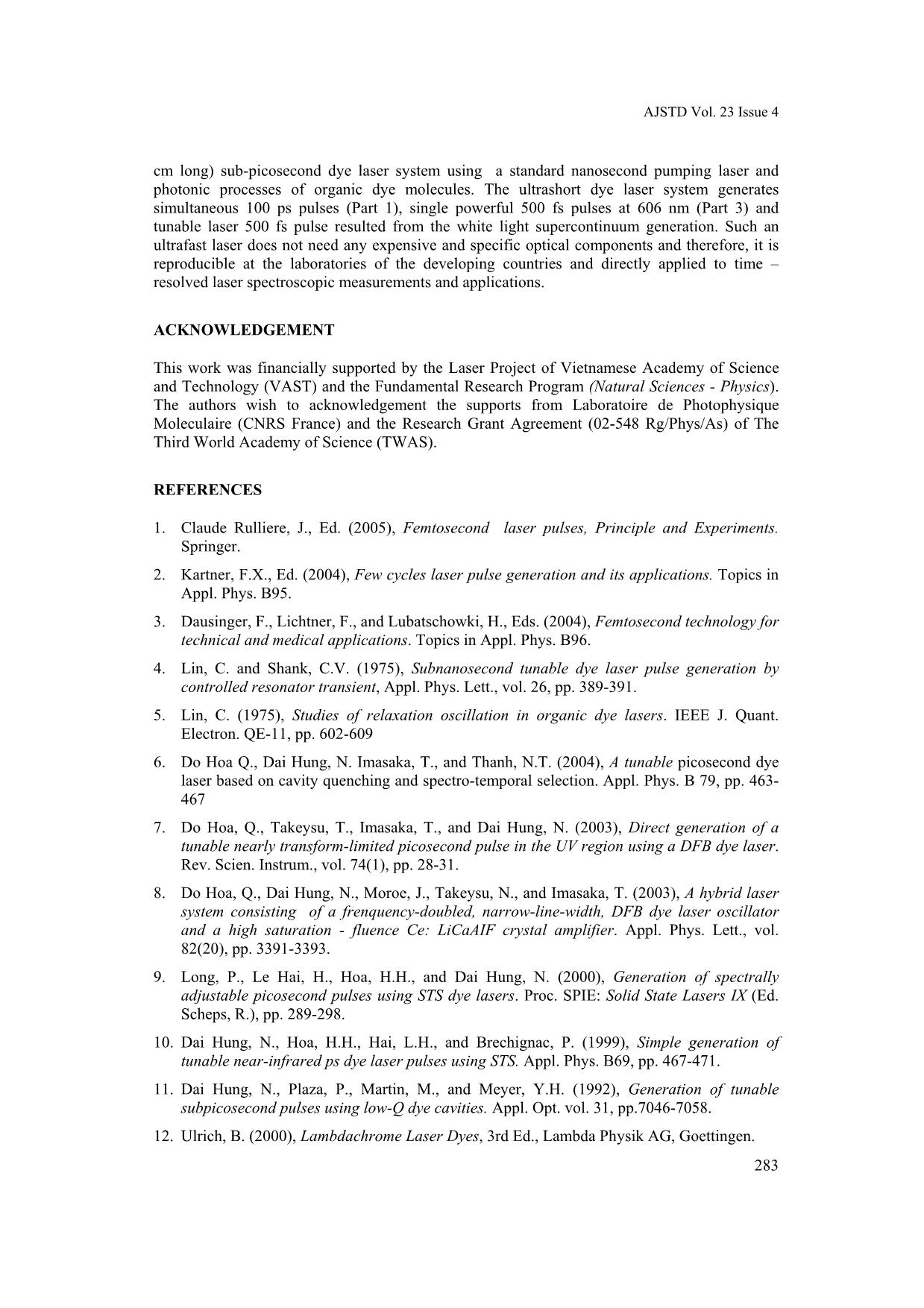
Trang 9
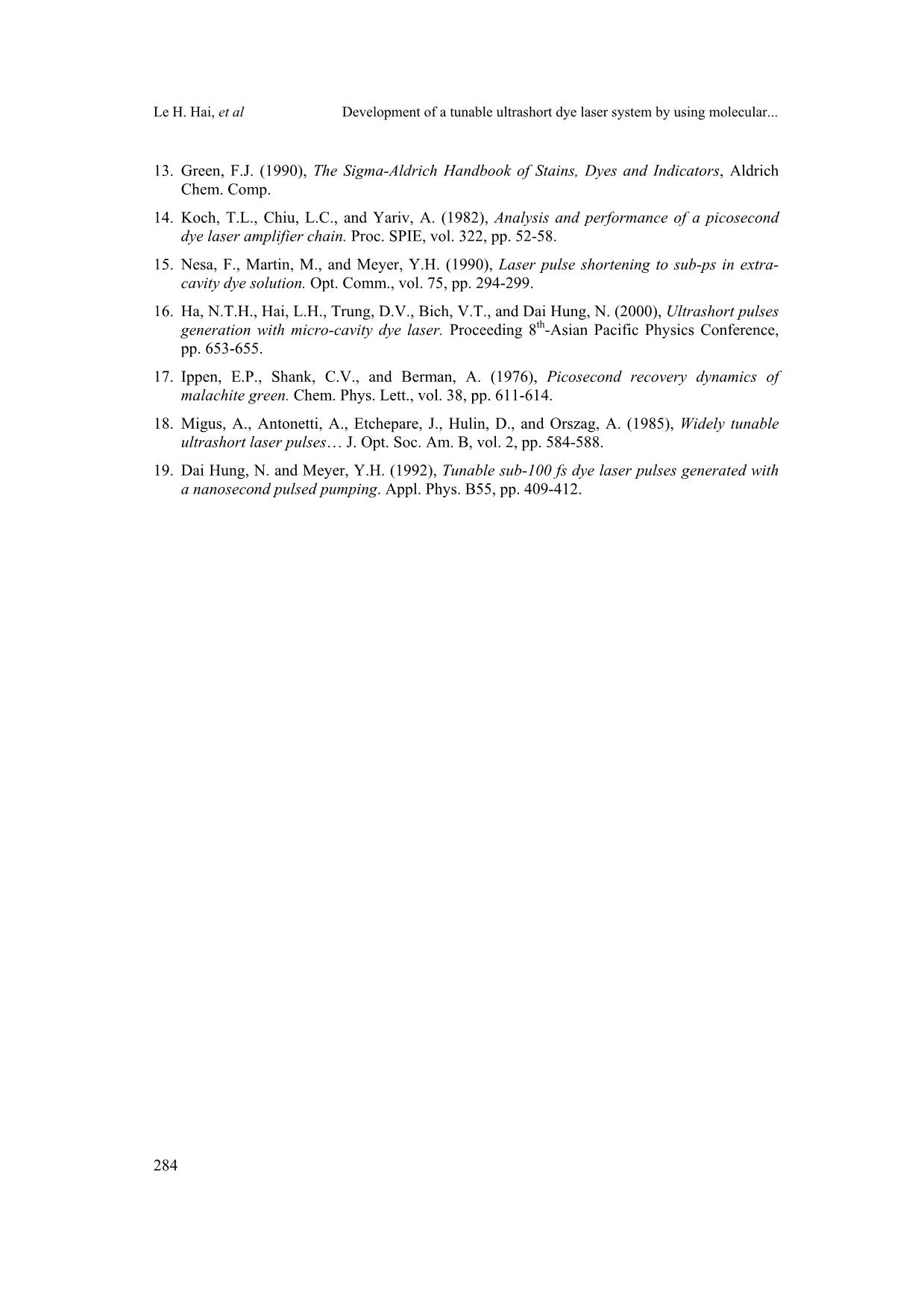
Trang 10
Tóm tắt nội dung tài liệu: Development of a tunable ultrashort dye laser system by using molecular photonic processes and a nanosecond pumping laser
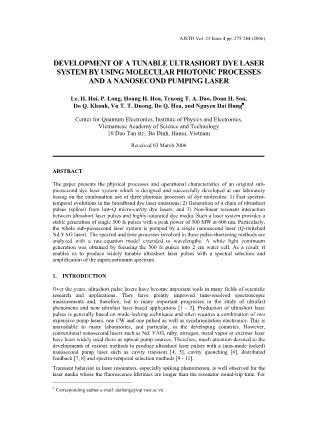
ndard 1 cm × 1 cm spectroscopic cell (DL- type Hellma), filled with solution of Rhodamine 6G in ethanol (4.10-4 M/l) and is transversally pumped at 10 times above threshold with 6 ns pump pulses. The dye cell constitutes a short and low-Q cavity with two un-coated side faces of 0.04 reflectivity. Figure 2 shows the spectral evolution in the broadband laser emission from such a dye laser by solving the rate equations extended to wavelengths [9 - 11] and using the dye molecular parameters [12, 13]: 1 1 0 1 1 1 NINII t N n i iei n i iaipap ⎟⎠ ⎞⎜⎝ ⎛ +−⎟⎠ ⎞⎜⎝ ⎛ += ∑∑ == στσσ∂ ∂ ( )[ ] 1012 NAT I lNN t I i i iaiei i +−−= ασσ∂ ∂ It should be noted that on the short-wavelength wing of the broadband laser spectrum, the laser oscillation lasts inherently for a short duration. This single short pulse can be obtained by a simple spectral se lec t ion . In practice, therefore, the STS method is to select, with an extra cavity filter, a spectral narrow band on the short-wavelength wing of the broadband laser emission from a short and low-Q dye laser oscillator. Its STS laser output is single short pulses, for current dyes, of less than 100 ps [9 - 11]. In our laser, the STS dye laser (Part 1- Fig. 1) is pumped by 6 ns pulses at 532 nm from the Nd:YAG laser. The broadband laser emission from O1 dye cell (Rh6G /ethanol solution, 4.10-4 M/l) is collimated and directed to a small grating G (600 grooves/mm, 1 cm × 2 cm), the second order diffracted beam is imaged and a spectral narrow band is selected with slit S of 0.3 mm wide. By that way, single short laser pulses of 75 ps and an energy of 15 nJ are produced. Le H. Hai, et al Development of a tunable ultrashort dye laser system by using molecular... 278 Sequently, the pulse is amplified in a 6-pass amplifier to 30 µJ. The amplifier used a bow-tie configuration with 1-mm thick dye cell (Rh6G/ethanol solution, 3.10-4 M/l, circulated). Such a STS dye laser generated single laser pulses of 75 ps (Fig. 3, assuming sech2 pulse shape), an pulse energy of 30 µJ at 562 nm and a spectral linewidth of 0.5 nm (FWHM). Fig. 3: Autocorrelation trace of output pulses from the Part 1 We have successfully operated the picosecond STS laser with different dyes (in liquid and solid state matrices) pumped at 355 nm or 532 nm by the third and second harmonic generation of the Nd:YAG laser in order to produce picosecond dye laser pulses (< 100 ps) spectrally adjustable from 386 nm to 860 nm [9 - 11]. In comparison with the mode-locked lasers that are used as optical pumping sources, these STS picosecond dye lasers have advantages such as compactness (25 cm × 35 cm), ease of operation, economy, possible use of many pump wavelengths and tunability of the output wavelength. 2.2 Part 2: Generation of subpicosecond pulses Part 2 is to convert the 75 ps pulses generated from the STS dye laser into sub-picosecond laser pulses (Fig. 1). For this purpose, we used an ultralow-Q micro-cavity dye laser (MC) to produce a chain of ultrashort pulses (spikes) and the highly- saturated dye amplifier and absorber for extra-microcavity pulse treatment. The ultralow-Q micro-cavity dye laser (MC) is made of two wedged silica plates (preferably wedged to avoid parasitic reflection) pressed together with a very thin layer of solution of Rhodamine 640/methanol (3.10-2 M/l). Similar small-path cells are commercially available (e.g., Hellma). MC is pumped longitudinally by the at 562 nm, 75 ps pulse that emitted from Part 1 through a 10 cm focal length lens. Applying the same type of calculations of the rate equation system extended to wavelengths, we have computed the spectral evolution of the laser emissions from the 50 µm low-Q dye laser cavity, as shown in Fig. 4, which is a chain of sub-picosecond pulses (spikes). Such a pulse structure well presents the spiking phenomenon in the laser relaxation oscillations of an ultralow-Q ultrashort laser cavity and, in particular, that well agrees with experimental one measured by a streak camera (Fig. 5). The MC laser emission spectrum could be changed by translation or rotation the MC in respect to the pumping beam axis (Fig. 4b). AJSTD Vol. 23 Issue 4 279 a) b) Fig. 4: Laser emission from a 50 µm microcavity filled with Rh640/methanol (C = 3.10-2 M/l) pumped by Gaussian shape pulses of 100 ps at 6 times above laser threshold. a) MC is perpendicular to the pumping beam; b) MC is tilted 40 Fig. 5: Typical emission from a 25 µm cavity In order to obtain single ultrashort laser pulses by extra-micro cavity pulse treatment, the micro cavity dye laser (MC) is followed by a multi-pass saturable dye amplifier (MSA), its configuration is similar to MPA in Part 1 and the Rh640/ethanol solution (3.10-4 M/l) is used as a gain medium. Because of highly-saturated gain, this amplifier functions mainly as a pulse shaper, the chain of spikes is strongly distorted pass by pass and the first spike becomes dominant in the amplified pulse chain. This pulse-shaping process is demonstrated by solving the following rate equation for gain G at each pass [14 - 16] and the results obtained are presented in Fig. 6. This pulse-shaping process is still effective until the sixth pass, that is why a six - pass saturable amplifier (MSA) configuration was used in our laser. ( ) ( ) ( )[ ] [ ]NLGGGtIGettIGv dt dG ass NL pases στσσ γκ +−−−−∆++= − ln11)(1)( 00 Le H. Hai, et al Development of a tunable ultrashort dye laser system by using molecular... 280 Fig. 6: a) The input signal; b) – h) Evolution of gain (dotted curves) and amplified signal intensity (solid curves) after each pass. (the left vertical axis: Signal intensity [photons.cm-2.s-1]; The right vertical axis: Gain) The distorted output from MSA is focused into a saturable absorber (SA). Due to absorption saturation, the first spike is isolated from the chain of spikes and well passed SA. This provides us single sub-picosecond pulses. Fig. 7 presents the computation results for this pulse isolation process using a 1 mm dye cell filled with Malachite Green (MG) in methanol as a saturable absorber [17]. As analyzed in [11], suitable selection of concentration, pumping energy and input signal intensity for MSA and SA is a critical task due to their significant influences on pulse distortion. In our laser system, the 25 µm MC is used and filled with Rh640/methanol solution (2.10-2 M/l) and pumped by 75 ps pulses from Part 1. The MC laser output is collimated and sent to MSA (Rh640/ethanol solution, 3.10-4 M/l). MSA is pumped semi-longitudinally by pulses of 6 ns (FWHM) and 3 mJ at 532 nm from the Nd:YAG laser. The synchronization between the signal and the pump pulses is performed by an optical delay line. The MSA output is of ~30 µJ with an amplified spontaneous emission energy of ~ 50%. Adequate focusing of the MSA output pulse AJSTD Vol. 23 Issue 4 281 into the Malachite Green saturable absorber cell (5.10-4 M/l) wipes out the spike tail and a single ultrashort spike - the first strongest one of ~1 µJ is obtained. Fig. 7: Action of saturable absorber in extracting single sub-ps pulses. a) output; b) input; c) optical density of absorber 2.3 Part 3: Power amplifier The single ultrashort laser pulse at 606 nm from Part 2 is amplified with three double-pass 1 cm amplifiers containing a circulated Rh640/ethanol solution (5.10-5 M/l). The second and the third amplifiers are separated by a 1 mm Malachite Green saturable absorber cell in order to eliminate ASE. The pumping pulse energies for the amplifiers are of 8, 10 and 10 mJ, respectively. Such a power amplification chain provides an amplifying factor of more than 100 that led to the output pulse energy of ~125 µJ. The pulse duration is measured with the autocorrelator which used a non-collinear second harmonic generation with a BBO nonlinear crystal. The autocorrelation traces (Fig. 8) are corresponded to two positions of the Malachite Green saturable absorber (SA). In both cases, output pulse duration is about 500 fs assuming the sech2 pulse shape. However, the obtained autocorrelation traces present the different actions of extra-cavity pulse treatment. For example, as the saturable absorber is at the tightly focused position of the input beam, the output could be multiple pulses corresponding to the enlargement of the autocorrelation trace on its foot (Fig. 8a). The trace in Fig. 8b corresponds to the shift of the saturable absorber 0.3 mm away from the previous position. At this position, the MG absorber is only saturated with the first and strongest spike, therefore, the output is the single pulses. In brief, at the output of Part 3 we have obtained the single dye laser pulses of ~500 fs in duration, 125 µJ in energy at 606 nm. Le H. Hai, et al Development of a tunable ultrashort dye laser system by using molecular... 282 a) b) Fig. 8: Autocorrelation traces of output laser pulses from Part 3 3. SUPERCONTINUUM GENERATION AND WIDELY TUNABLE 500 FS PULSE A white light continuum is generated by focusing the ~100 µJ - 500 fs pulse into 2 cm water cell with a 10 cm focal length lens. As a result of self-phase modulation, the supercontinuum spectrum is obtained, as presented in Fig. 9, and strongly broadened to cover over the UV, visible and near infrared spectral regions. The energy of the white light supercontinuum varies by 4 orders of magnitude from the central wavelength to both continuum wings (345 and 860 nm). Significantly, the white light continuum generation enables one to produce widely tunable ultrashort pulses. By selecting a narrow band in the supercontinuum with an interferential filters of 1 nm FWHM bandwidth, we have produced nearly transform-limited subpicosecond pulses at a desired wavelength. Furthermore, widely and continuous tunability of ultrashort laser pulse from 540 nm to 720 nm is obtained by using suitable spectral filters and several dyes in dye amplifiers pumped at 532 nm. With the 355 nm pumping by the third harmonic of the same Nd:YAG laser, the system generates tunable sub-picosecond pulses between 540 nm to 450 nm with Coumarin dyes. Detail presentations of spectral selection and amplification of a desire spectral band from the supercontinuum spectrum were showed [18, 19]. Fig. 9: Supercontinuum Spectrum of 500 fs laser pulse after passing through a water cell of 2 cm long 4. CONCLUSION In this paper we have presented a simple, low-cost and relatively compact (80 cm wide × 100 AJSTD Vol. 23 Issue 4 283 cm long) sub-picosecond dye laser system using a standard nanosecond pumping laser and photonic processes of organic dye molecules. The ultrashort dye laser system generates simultaneous 100 ps pulses (Part 1), single powerful 500 fs pulses at 606 nm (Part 3) and tunable laser 500 fs pulse resulted from the white light supercontinuum generation. Such an ultrafast laser does not need any expensive and specific optical components and therefore, it is reproducible at the laboratories of the developing countries and directly applied to time – resolved laser spectroscopic measurements and applications. ACKNOWLEDGEMENT This work was financially supported by the Laser Project of Vietnamese Academy of Science and Technology (VAST) and the Fundamental Research Program (Natural Sciences - Physics). The authors wish to acknowledgement the supports from Laboratoire de Photophysique Moleculaire (CNRS France) and the Research Grant Agreement (02-548 Rg/Phys/As) of The Third World Academy of Science (TWAS). REFERENCES 1. Claude Rulliere, J., Ed. (2005), Femtosecond laser pulses, Principle and Experiments. Springer. 2. Kartner, F.X., Ed. (2004), Few cycles laser pulse generation and its applications. Topics in Appl. Phys. B95. 3. Dausinger, F., Lichtner, F., and Lubatschowki, H., Eds. (2004), Femtosecond technology for technical and medical applications. Topics in Appl. Phys. B96. 4. Lin, C. and Shank, C.V. (1975), Subnanosecond tunable dye laser pulse generation by controlled resonator transient, Appl. Phys. Lett., vol. 26, pp. 389-391. 5. Lin, C. (1975), Studies of relaxation oscillation in organic dye lasers. IEEE J. Quant. Electron. QE-11, pp. 602-609 6. Do Hoa Q., Dai Hung, N. Imasaka, T., and Thanh, N.T. (2004), A tunable picosecond dye laser based on cavity quenching and spectro-temporal selection. Appl. Phys. B 79, pp. 463- 467 7. Do Hoa, Q., Takeysu, T., Imasaka, T., and Dai Hung, N. (2003), Direct generation of a tunable nearly transform-limited picosecond pulse in the UV region using a DFB dye laser. Rev. Scien. Instrum., vol. 74(1), pp. 28-31. 8. Do Hoa, Q., Dai Hung, N., Moroe, J., Takeysu, N., and Imasaka, T. (2003), A hybrid laser system consisting of a frenquency-doubled, narrow-line-width, DFB dye laser oscillator and a high saturation - fluence Ce: LiCaAIF crystal amplifier. Appl. Phys. Lett., vol. 82(20), pp. 3391-3393. 9. Long, P., Le Hai, H., Hoa, H.H., and Dai Hung, N. (2000), Generation of spectrally adjustable picosecond pulses using STS dye lasers. Proc. SPIE: Solid State Lasers IX (Ed. Scheps, R.), pp. 289-298. 10. Dai Hung, N., Hoa, H.H., Hai, L.H., and Brechignac, P. (1999), Simple generation of tunable near-infrared ps dye laser pulses using STS. Appl. Phys. B69, pp. 467-471. 11. Dai Hung, N., Plaza, P., Martin, M., and Meyer, Y.H. (1992), Generation of tunable subpicosecond pulses using low-Q dye cavities. Appl. Opt. vol. 31, pp.7046-7058. 12. Ulrich, B. (2000), Lambdachrome Laser Dyes, 3rd Ed., Lambda Physik AG, Goettingen. Le H. Hai, et al Development of a tunable ultrashort dye laser system by using molecular... 284 13. Green, F.J. (1990), The Sigma-Aldrich Handbook of Stains, Dyes and Indicators, Aldrich Chem. Comp. 14. Koch, T.L., Chiu, L.C., and Yariv, A. (1982), Analysis and performance of a picosecond dye laser amplifier chain. Proc. SPIE, vol. 322, pp. 52-58. 15. Nesa, F., Martin, M., and Meyer, Y.H. (1990), Laser pulse shortening to sub-ps in extra- cavity dye solution. Opt. Comm., vol. 75, pp. 294-299. 16. Ha, N.T.H., Hai, L.H., Trung, D.V., Bich, V.T., and Dai Hung, N. (2000), Ultrashort pulses generation with micro-cavity dye laser. Proceeding 8th-Asian Pacific Physics Conference, pp. 653-655. 17. Ippen, E.P., Shank, C.V., and Berman, A. (1976), Picosecond recovery dynamics of malachite green. Chem. Phys. Lett., vol. 38, pp. 611-614. 18. Migus, A., Antonetti, A., Etchepare, J., Hulin, D., and Orszag, A. (1985), Widely tunable ultrashort laser pulses J. Opt. Soc. Am. B, vol. 2, pp. 584-588. 19. Dai Hung, N. and Meyer, Y.H. (1992), Tunable sub-100 fs dye laser pulses generated with a nanosecond pulsed pumping. Appl. Phys. B55, pp. 409-412.
File đính kèm:
development_of_a_tunable_ultrashort_dye_laser_system_by_usin.pdf