Depth estimation of the absorbing structure in a slab turbid medium using point spread function
Nowadays, transillumination imaging is more popular used in the medical field with the development of the vein finder application and also the non-invasive diagnosis applications. The less absorption of those main chromophores (melanin, oxy-hemoglobin, deoxy-hemoglobin, and water)
of biological tissue within the near-infrared range (700 - 1200 nm) causes the relatively high transmission of the near-infrared light through biological tissue. They give us an "optical window" to seethrough the biological tissue non-invasively. Because of the high absorption of oxy-hemoglobin,
deoxy-hemoglobin within the near-infrared range in comparison with these other parts in tissue,
we can able to obtain a two dimensional (2D) transillumination image of the internal absorption
structure such as blood vessel network, liver structure. in the body non-invasively. Using the lightemitting diode (LED)'s array to illuminate the human arm and using a low-cost camera to capture
the image, we could obtain the blood vessel network image of the human arm. The captured
image is blurred and difficult to distingue the structure inside. Because the camera captured the
blood vessel network shadow that diffused on the skin surface. Professor Koichi Shimizu devised
the depth-dependent point spread function (PSF) to describe the scattering effect from a light point
source to the observation surface of a slab diffused medium by applying the diffusion approximation. By suppressing the scattering effect successfully, we could restore the clear image from the
blurred image by de-convolution with the appropriated PSF. With our proposed technique, we
could reconstruct the absorbing structures such as the kidney and liver in biological tissue. Using
the restored images from different angles of view, we could reconstruct the cross-sectional images
and three-dimensional image of the absorbing structures in an animal's abdomen. However, the
depth information of the absorbing-structure was required in practice to calculate the appropriate
PSF. Therefore, in order to make this method more practical, the depth information is crucial. In
this paper, we proposed the novel techniques for estimating the parameters of absorbing structure (depth, center location, and width) in the turbid medium by using the convolution and deconvolution operations with the devised PSF. Firstly, by observing images with two-wavelength selected at which the absorption and scattering properties of the medium are different. While changing the depth step-by-step, two convoluted images were compared after convolving the captured
image at one of the wavelengths with the PSF calculated by using the absorption and scattering
coefficients from another wavelength. We can obtain the correct depth that gives the minimum
difference between the two convoluted images. Secondly, we proposed the technique to estimate
the depth information from a single transillumination image in order to make this method more
practical. Finally, the absorbing structure image can be restored by suppressing the scattering effect. The proposed techniques were validated and examined not only in the simulation but also in
the experiment
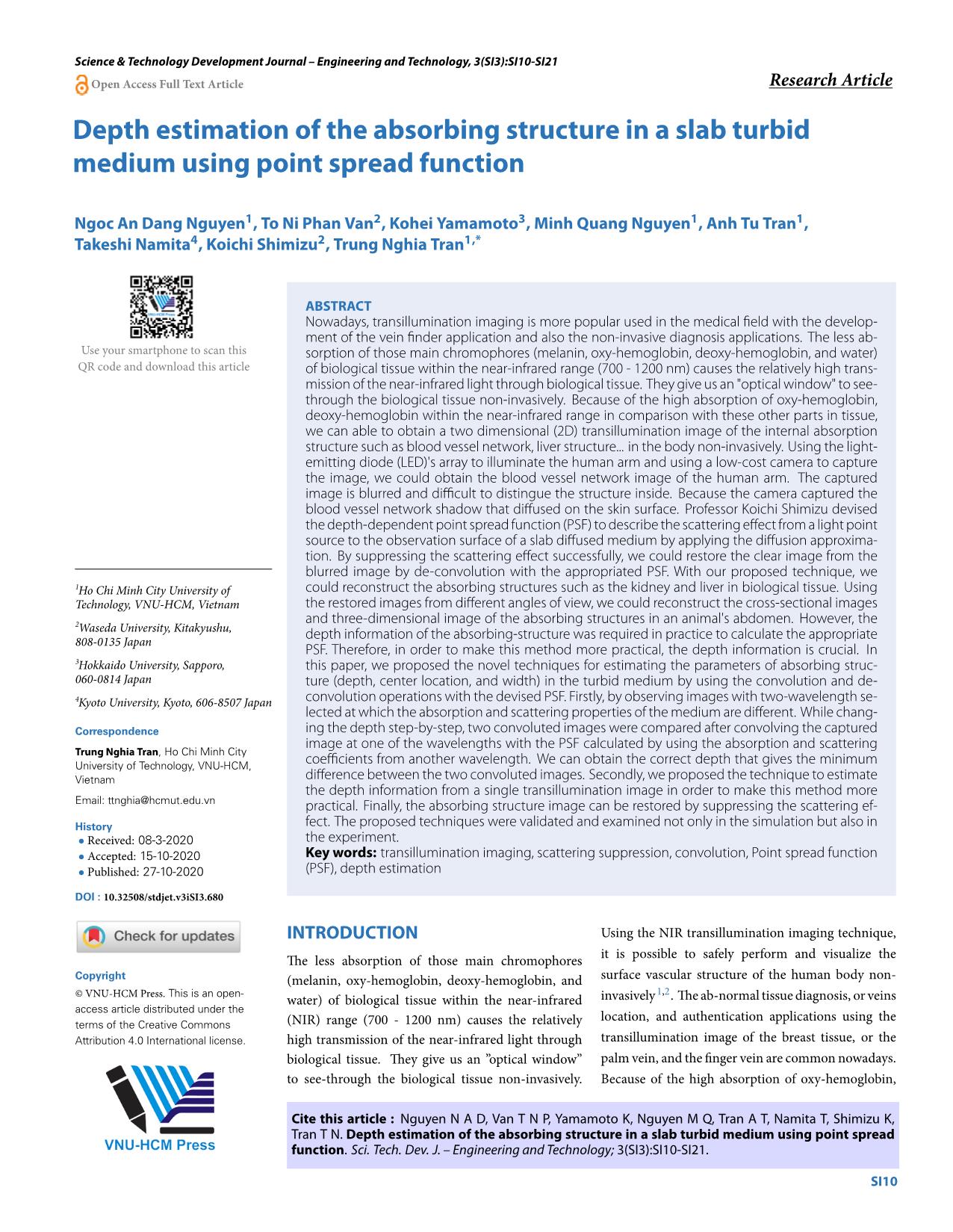
Trang 1
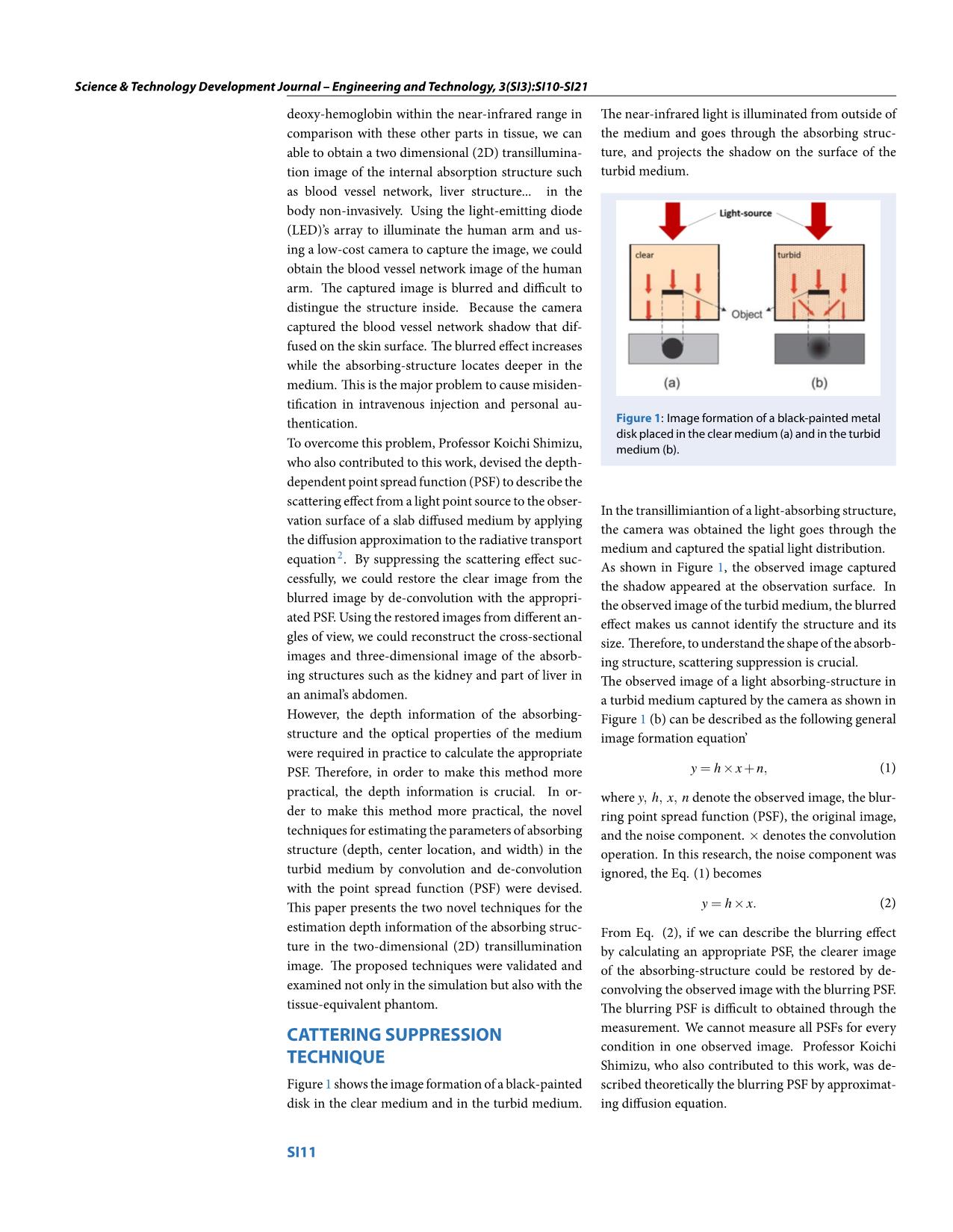
Trang 2
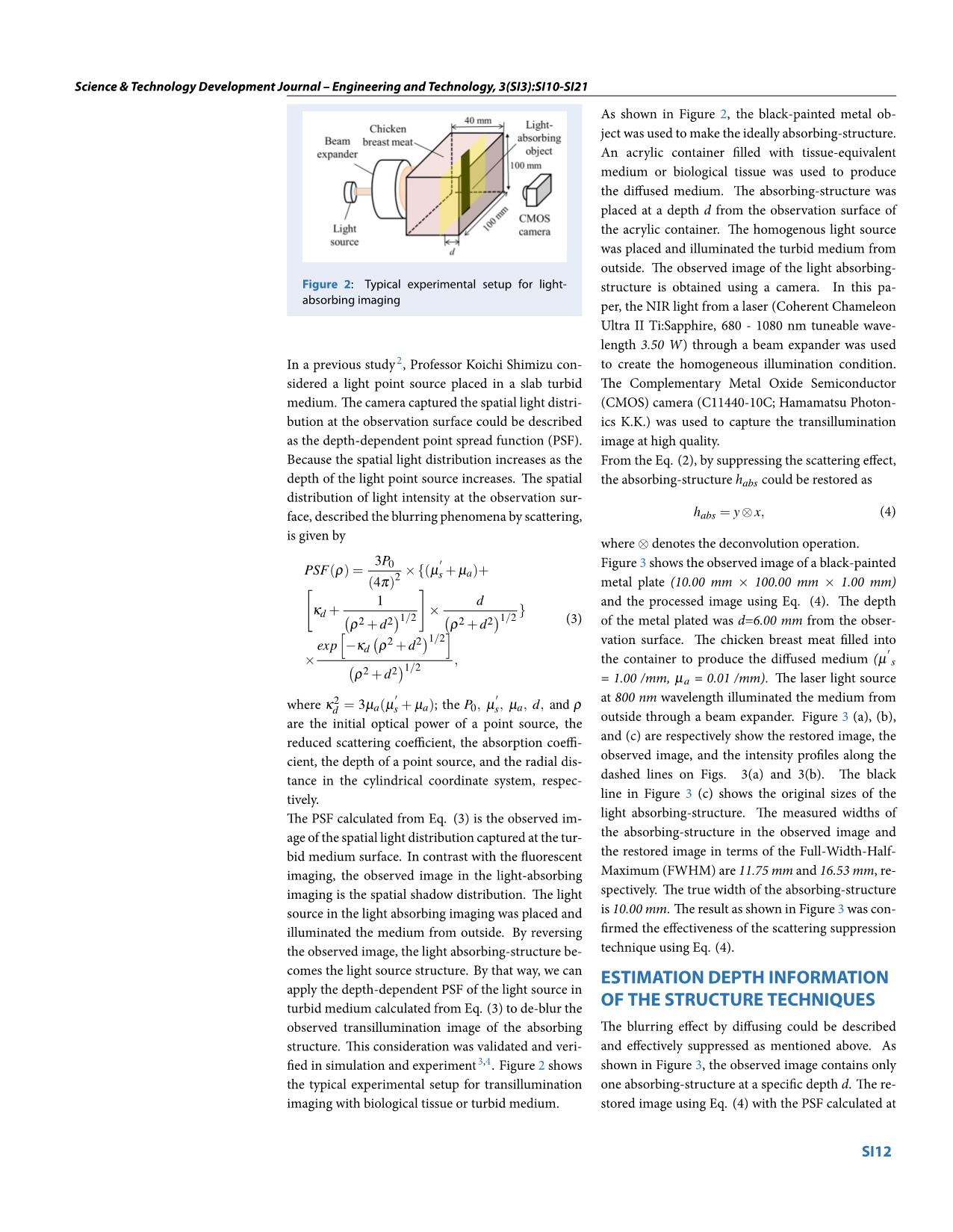
Trang 3
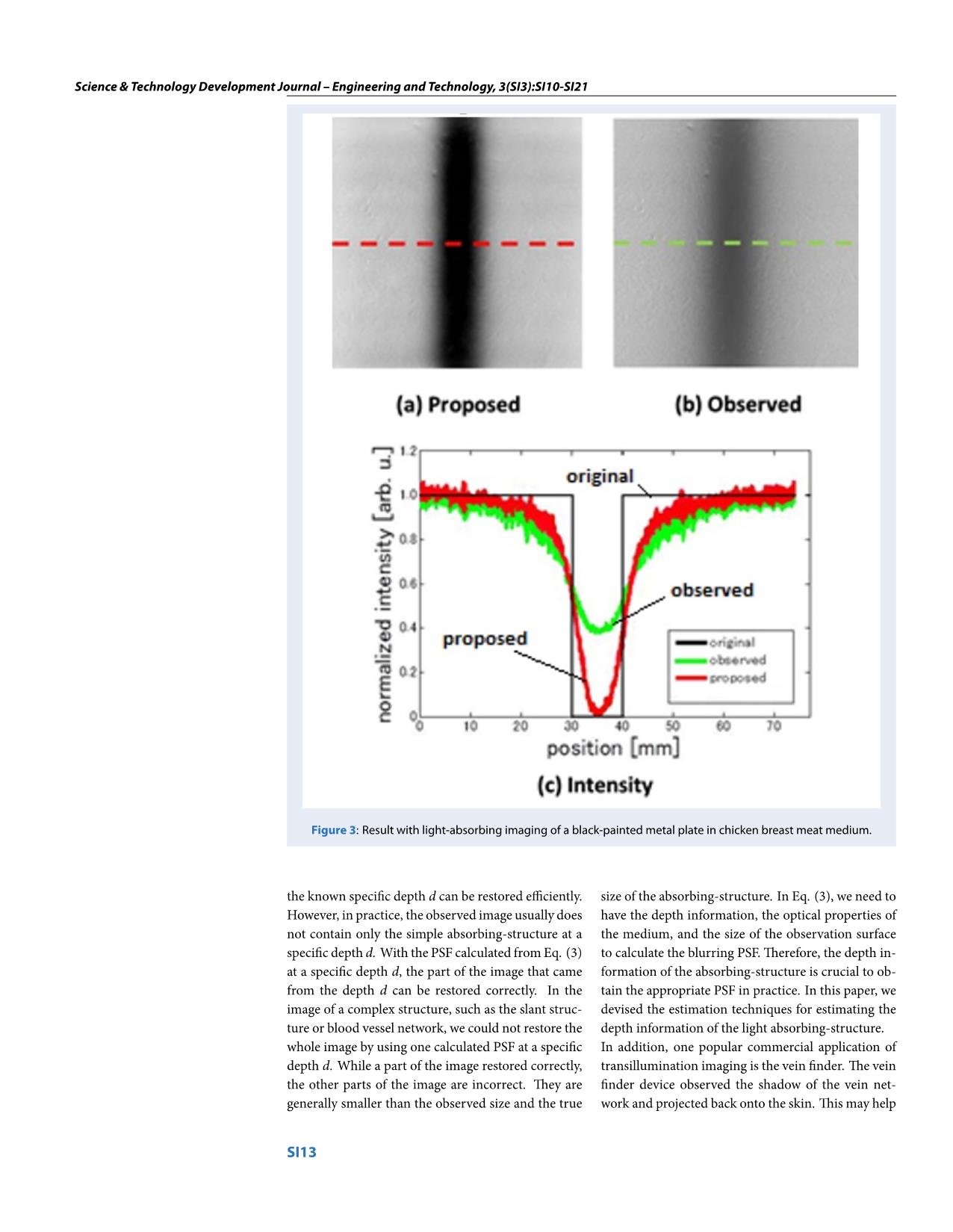
Trang 4
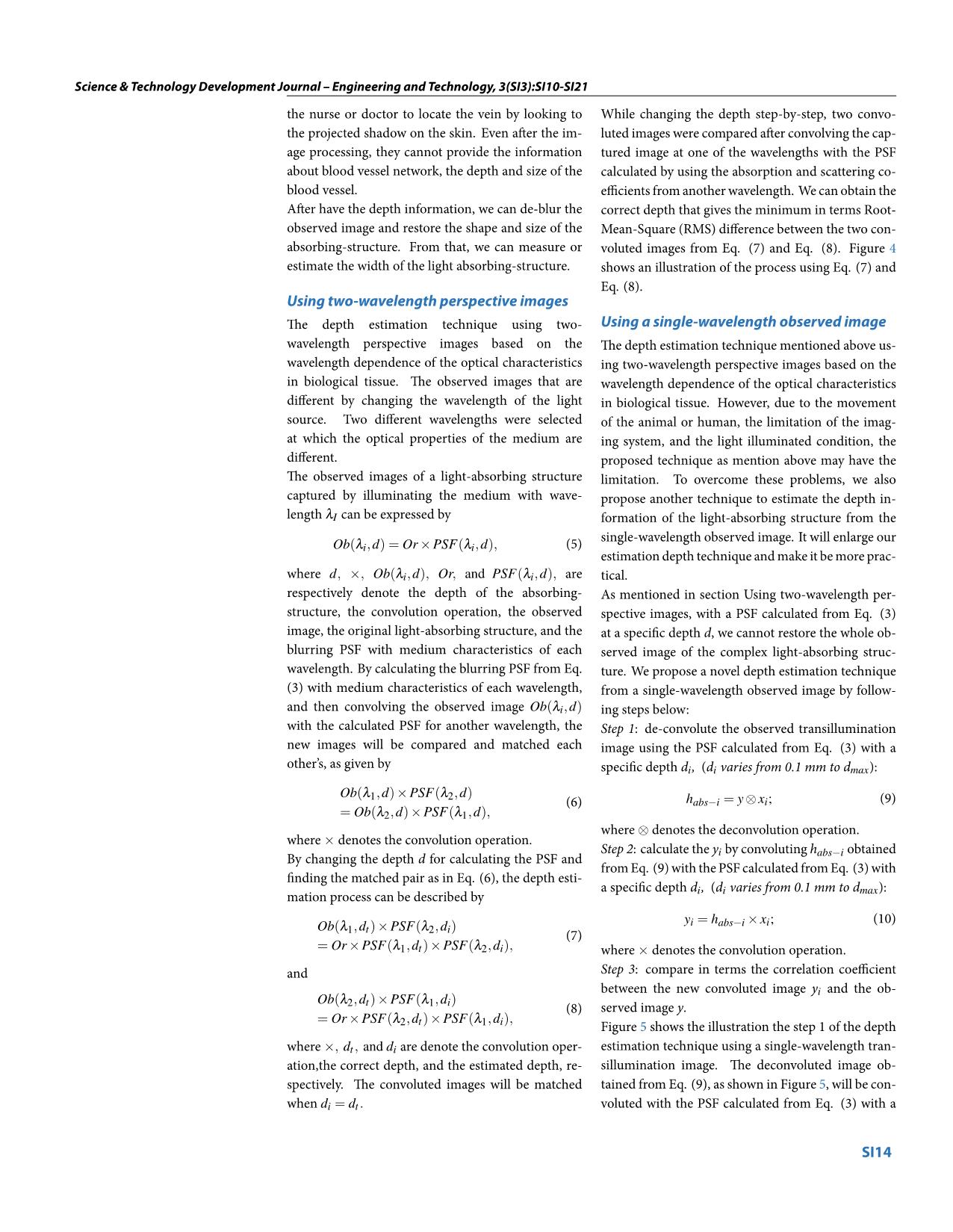
Trang 5
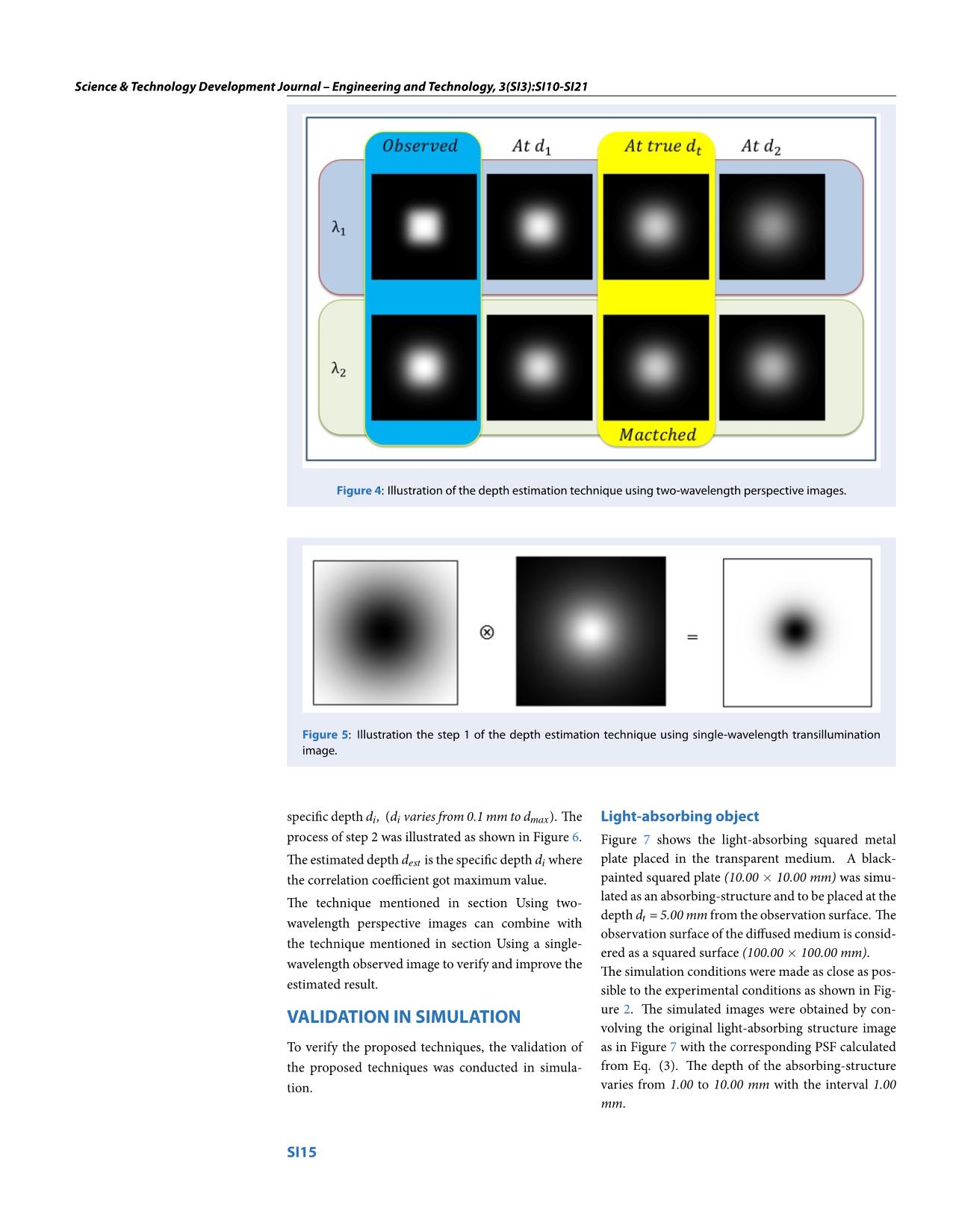
Trang 6
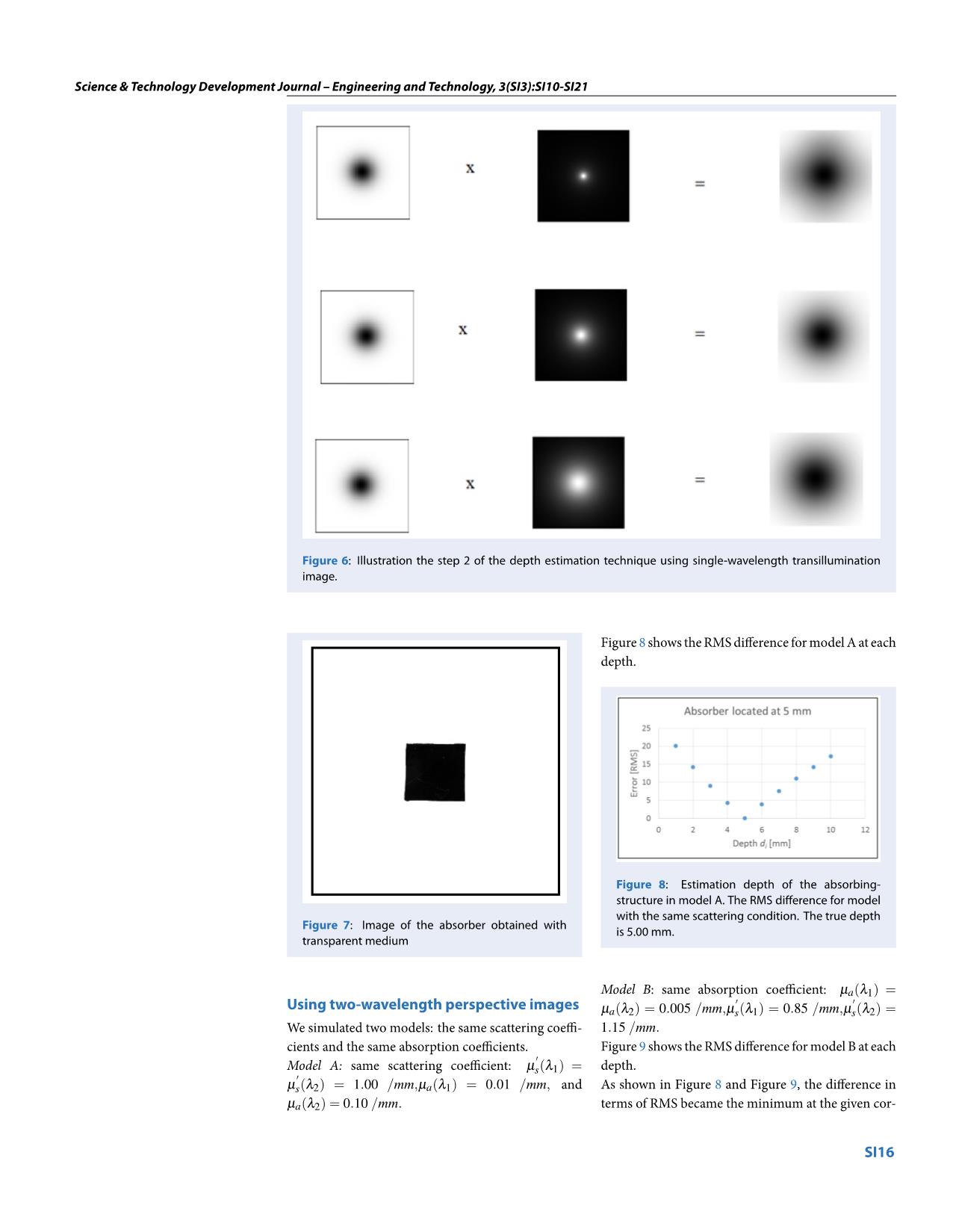
Trang 7
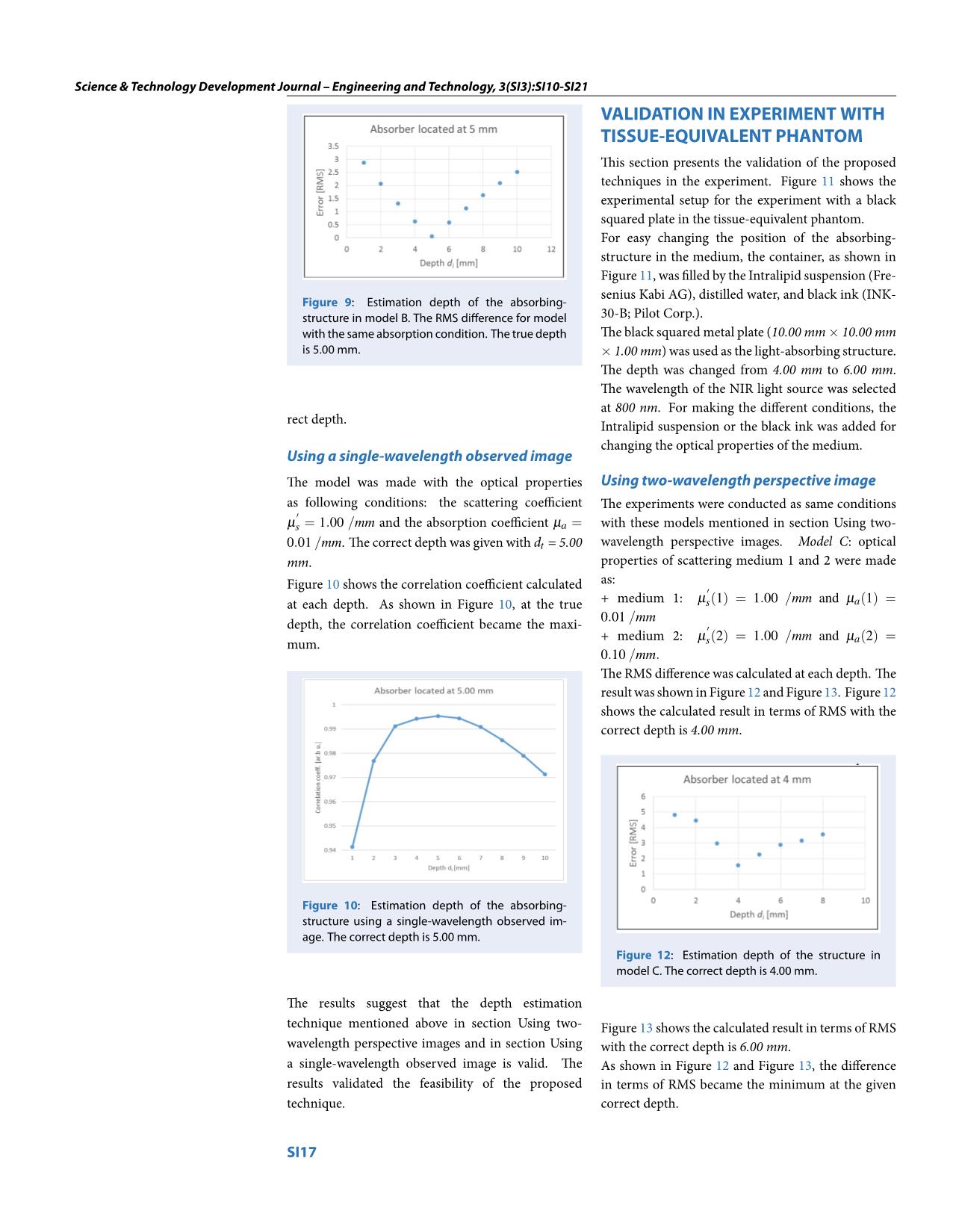
Trang 8
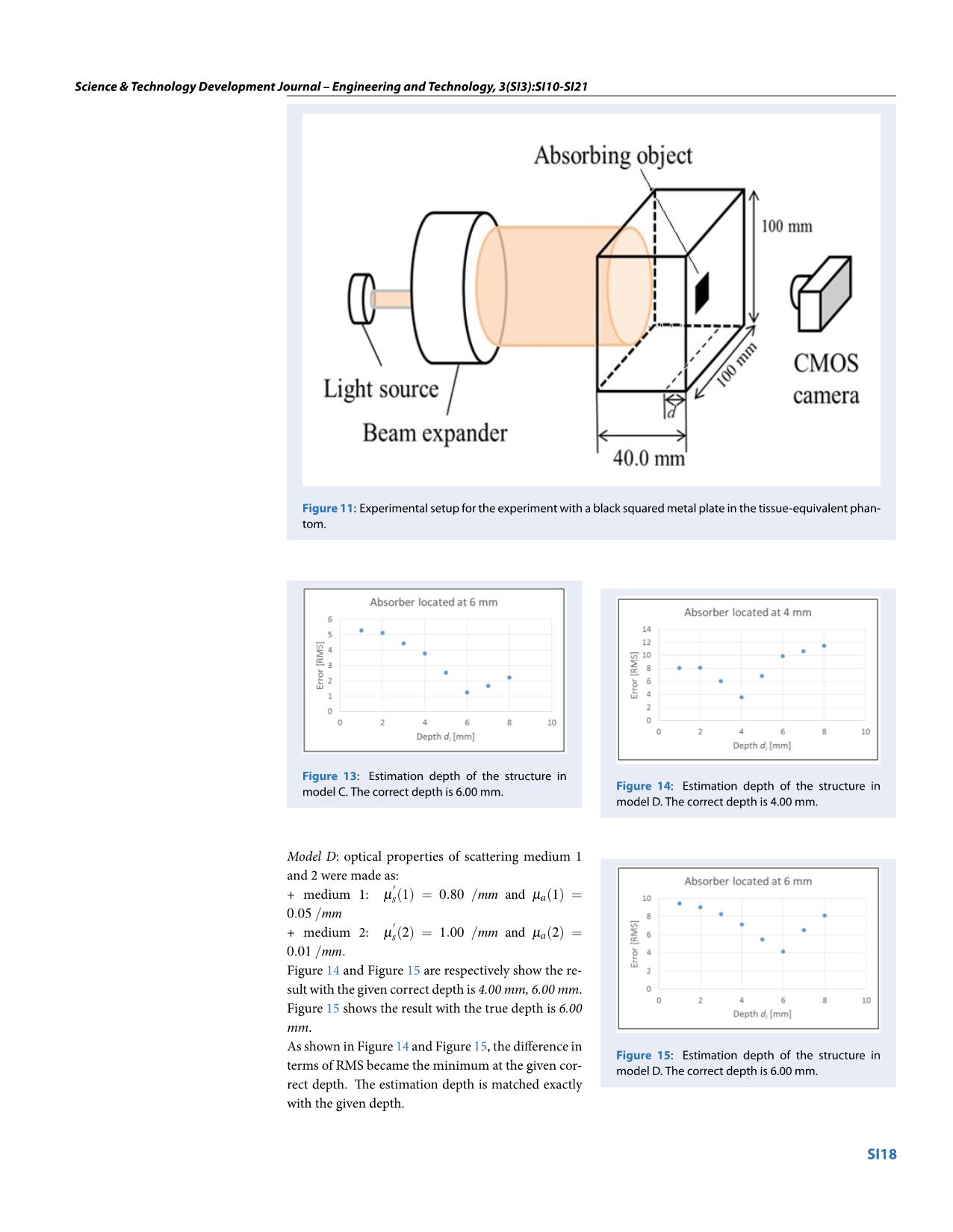
Trang 9
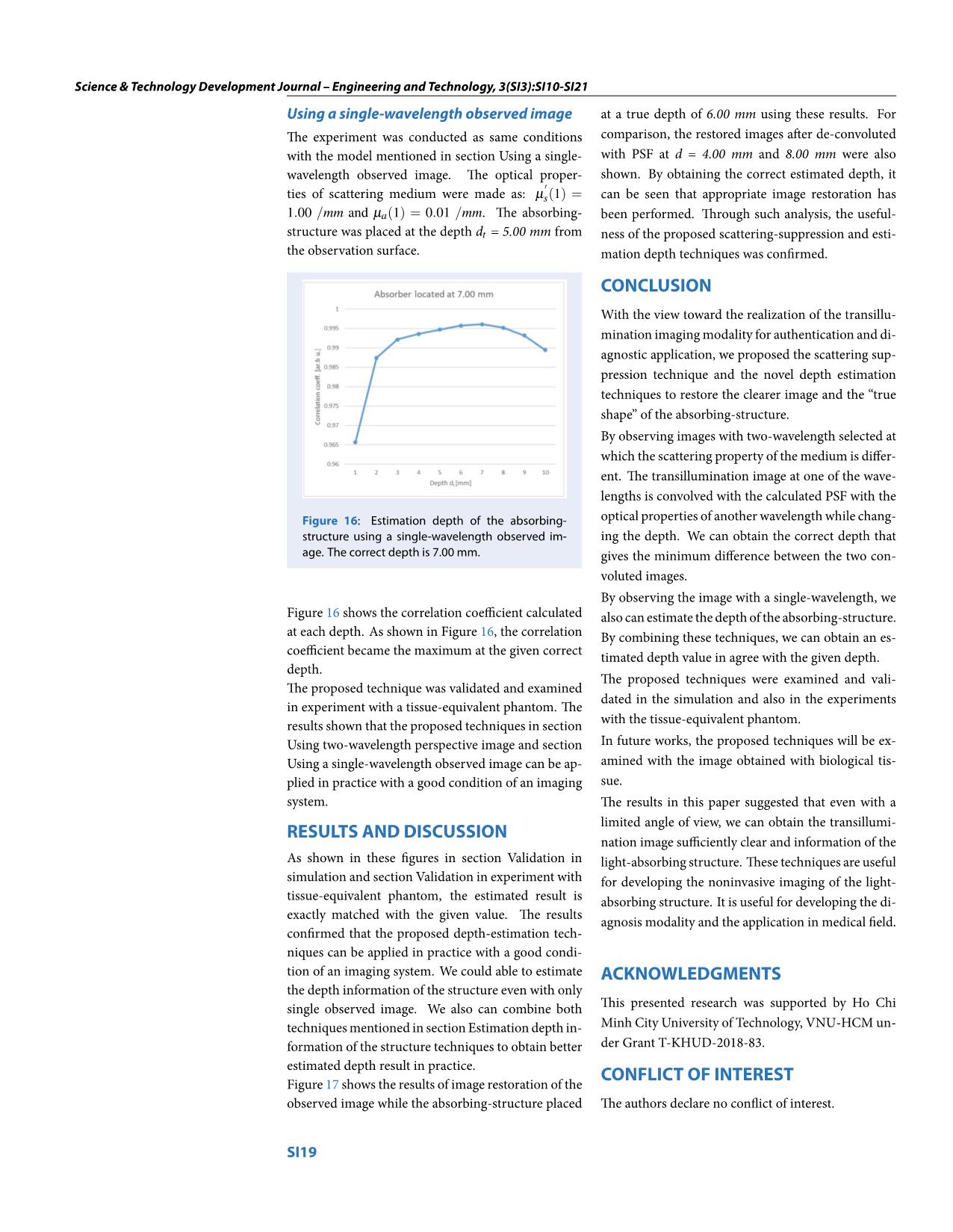
Trang 10
Tải về để xem bản đầy đủ
Tóm tắt nội dung tài liệu: Depth estimation of the absorbing structure in a slab turbid medium using point spread function
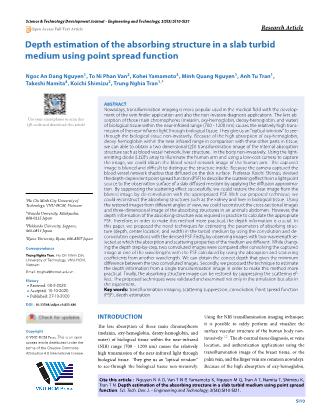
g the cap- tured image at one of the wavelengths with the PSF calculated by using the absorption and scattering co- efficients from anotherwavelength. We can obtain the correct depth that gives the minimum in terms Root- Mean-Square (RMS) difference between the two con- voluted images from Eq. (7) and Eq. (8). Figure 4 shows an illustration of the process using Eq. (7) and Eq. (8). Using a single-wavelength observed image The depth estimation technique mentioned above us- ing two-wavelength perspective images based on the wavelength dependence of the optical characteristics in biological tissue. However, due to the movement of the animal or human, the limitation of the imag- ing system, and the light illuminated condition, the proposed technique as mention above may have the limitation. To overcome these problems, we also propose another technique to estimate the depth in- formation of the light-absorbing structure from the single-wavelength observed image. It will enlarge our estimation depth technique andmake it bemore prac- tical. As mentioned in section Using two-wavelength per- spective images, with a PSF calculated from Eq. (3) at a specific depth d, we cannot restore the whole ob- served image of the complex light-absorbing struc- ture. We propose a novel depth estimation technique from a single-wavelength observed image by follow- ing steps below: Step 1: de-convolute the observed transillumination image using the PSF calculated from Eq. (3) with a specific depth di, (di varies from 0.1 mm to dmax): habs i = y xi; (9) where denotes the deconvolution operation. Step 2: calculate the yi by convoluting habs i obtained fromEq. (9)with the PSF calculated fromEq. (3)with a specific depth di, (di varies from 0.1 mm to dmax): yi = habs i xi; (10) where denotes the convolution operation. Step 3: compare in terms the correlation coefficient between the new convoluted image yi and the ob- served image y. Figure 5 shows the illustration the step 1 of the depth estimation technique using a single-wavelength tran- sillumination image. The deconvoluted image ob- tained from Eq. (9), as shown in Figure 5, will be con- voluted with the PSF calculated from Eq. (3) with a SI14 Science & Technology Development Journal – Engineering and Technology, 3(SI3):SI10-SI21 Figure 4: Illustration of the depth estimation technique using two-wavelength perspective images. Figure 5: Illustration the step 1 of the depth estimation technique using single-wavelength transillumination image. specific depth di, (di varies from 0.1 mm to dmax). The process of step 2 was illustrated as shown in Figure 6. The estimated depth dest is the specific depth di where the correlation coefficient got maximum value. The technique mentioned in section Using two- wavelength perspective images can combine with the technique mentioned in section Using a single- wavelength observed image to verify and improve the estimated result. VALIDATION IN SIMULATION To verify the proposed techniques, the validation of the proposed techniques was conducted in simula- tion. Light-absorbing object Figure 7 shows the light-absorbing squared metal plate placed in the transparent medium. A black- painted squared plate (10.00 10.00 mm) was simu- lated as an absorbing-structure and to be placed at the depth dt = 5.00mm from the observation surface. The observation surface of the diffusedmedium is consid- ered as a squared surface (100.00 100.00 mm). The simulation conditions were made as close as pos- sible to the experimental conditions as shown in Fig- ure 2. The simulated images were obtained by con- volving the original light-absorbing structure image as in Figure 7 with the corresponding PSF calculated from Eq. (3). The depth of the absorbing-structure varies from 1.00 to 10.00 mm with the interval 1.00 mm. SI15 Science & Technology Development Journal – Engineering and Technology, 3(SI3):SI10-SI21 Figure 6: Illustration the step 2 of the depth estimation technique using single-wavelength transillumination image. Figure 7: Image of the absorber obtained with transparent medium Using two-wavelength perspective images We simulated two models: the same scattering coeffi- cients and the same absorption coefficients. Model A: same scattering coefficient: m 0s(l1) = m 0s(l2) = 1:00 =mm;ma(l1) = 0:01 =mm; and ma(l2) = 0:10 =mm: Figure 8 shows theRMSdifference formodelA at each depth. Figure 8: Estimation depth of the absorbing- structure in model A. The RMS difference for model with the same scattering condition. The true depth is 5.00 mm. Model B: same absorption coefficient: ma(l1) = ma(l2) = 0:005 =mm;m 0 s(l1) = 0:85 =mm;m 0 s(l2) = 1:15 =mm: Figure 9 shows the RMSdifference formodel B at each depth. As shown in Figure 8 and Figure 9, the difference in terms of RMS became the minimum at the given cor- SI16 Science & Technology Development Journal – Engineering and Technology, 3(SI3):SI10-SI21 Figure 9: Estimation depth of the absorbing- structure in model B. The RMS difference for model with the same absorption condition. The true depth is 5.00 mm. rect depth. Using a single-wavelength observed image The model was made with the optical properties as following conditions: the scattering coefficient m 0s = 1:00 =mm and the absorption coefficient ma = 0:01 =mm. The correct depth was given with dt = 5.00 mm. Figure 10 shows the correlation coefficient calculated at each depth. As shown in Figure 10, at the true depth, the correlation coefficient became the maxi- mum. Figure 10: Estimation depth of the absorbing- structure using a single-wavelength observed im- age. The correct depth is 5.00 mm. The results suggest that the depth estimation technique mentioned above in section Using two- wavelength perspective images and in section Using a single-wavelength observed image is valid. The results validated the feasibility of the proposed technique. VALIDATION IN EXPERIMENTWITH TISSUE-EQUIVALENT PHANTOM This section presents the validation of the proposed techniques in the experiment. Figure 11 shows the experimental setup for the experiment with a black squared plate in the tissue-equivalent phantom. For easy changing the position of the absorbing- structure in the medium, the container, as shown in Figure 11, was filled by the Intralipid suspension (Fre- senius Kabi AG), distilled water, and black ink (INK- 30-B; Pilot Corp.). The black squaredmetal plate (10.00 mm 10.00 mm 1.00mm) was used as the light-absorbing structure. The depth was changed from 4.00 mm to 6.00 mm. The wavelength of the NIR light source was selected at 800 nm. For making the different conditions, the Intralipid suspension or the black ink was added for changing the optical properties of the medium. Using two-wavelength perspective image The experiments were conducted as same conditions with these models mentioned in section Using two- wavelength perspective images. Model C: optical properties of scattering medium 1 and 2 were made as: + medium 1: m 0s(1) = 1:00 =mm and ma(1) = 0:01 =mm + medium 2: m 0s(2) = 1:00 =mm and ma(2) = 0:10 =mm: TheRMS difference was calculated at each depth. The result was shown in Figure 12 andFigure 13. Figure 12 shows the calculated result in terms of RMS with the correct depth is 4.00 mm. Figure 12: Estimation depth of the structure in model C. The correct depth is 4.00 mm. Figure 13 shows the calculated result in terms of RMS with the correct depth is 6.00 mm. As shown in Figure 12 and Figure 13, the difference in terms of RMS became the minimum at the given correct depth. SI17 Science & Technology Development Journal – Engineering and Technology, 3(SI3):SI10-SI21 Figure 11: Experimental setup for the experiment with a black squaredmetal plate in the tissue-equivalent phan- tom. Figure 13: Estimation depth of the structure in model C. The correct depth is 6.00 mm. Model D: optical properties of scattering medium 1 and 2 were made as: + medium 1: m 0s(1) = 0:80 =mm and ma(1) = 0:05 =mm + medium 2: m 0s(2) = 1:00 =mm and ma(2) = 0:01 =mm: Figure 14 and Figure 15 are respectively show the re- sult with the given correct depth is 4.00mm, 6.00mm. Figure 15 shows the result with the true depth is 6.00 mm. As shown in Figure 14 and Figure 15, the difference in terms of RMS became the minimum at the given cor- rect depth. The estimation depth is matched exactly with the given depth. Figure 14: Estimation depth of the structure in model D. The correct depth is 4.00 mm. Figure 15: Estimation depth of the structure in model D. The correct depth is 6.00 mm. SI18 Science & Technology Development Journal – Engineering and Technology, 3(SI3):SI10-SI21 Using a single-wavelength observed image The experiment was conducted as same conditions with the model mentioned in section Using a single- wavelength observed image. The optical proper- ties of scattering medium were made as: m 0s(1) = 1:00 =mm and ma(1) = 0:01 =mm. The absorbing- structure was placed at the depth dt = 5.00 mm from the observation surface. Figure 16: Estimation depth of the absorbing- structure using a single-wavelength observed im- age. The correct depth is 7.00 mm. Figure 16 shows the correlation coefficient calculated at each depth. As shown in Figure 16, the correlation coefficient became the maximum at the given correct depth. The proposed technique was validated and examined in experiment with a tissue-equivalent phantom. The results shown that the proposed techniques in section Using two-wavelength perspective image and section Using a single-wavelength observed image can be ap- plied in practice with a good condition of an imaging system. RESULTS ANDDISCUSSION As shown in these figures in section Validation in simulation and section Validation in experiment with tissue-equivalent phantom, the estimated result is exactly matched with the given value. The results confirmed that the proposed depth-estimation tech- niques can be applied in practice with a good condi- tion of an imaging system. We could able to estimate the depth information of the structure even with only single observed image. We also can combine both techniquesmentioned in section Estimation depth in- formation of the structure techniques to obtain better estimated depth result in practice. Figure 17 shows the results of image restoration of the observed image while the absorbing-structure placed at a true depth of 6.00 mm using these results. For comparison, the restored images after de-convoluted with PSF at d = 4.00 mm and 8.00 mm were also shown. By obtaining the correct estimated depth, it can be seen that appropriate image restoration has been performed. Through such analysis, the useful- ness of the proposed scattering-suppression and esti- mation depth techniques was confirmed. CONCLUSION With the view toward the realization of the transillu- mination imagingmodality for authentication and di- agnostic application, we proposed the scattering sup- pression technique and the novel depth estimation techniques to restore the clearer image and the “true shape” of the absorbing-structure. By observing images with two-wavelength selected at which the scattering property of the medium is differ- ent. The transillumination image at one of the wave- lengths is convolved with the calculated PSF with the optical properties of another wavelength while chang- ing the depth. We can obtain the correct depth that gives the minimum difference between the two con- voluted images. By observing the image with a single-wavelength, we also can estimate the depth of the absorbing-structure. By combining these techniques, we can obtain an es- timated depth value in agree with the given depth. The proposed techniques were examined and vali- dated in the simulation and also in the experiments with the tissue-equivalent phantom. In future works, the proposed techniques will be ex- amined with the image obtained with biological tis- sue. The results in this paper suggested that even with a limited angle of view, we can obtain the transillumi- nation image sufficiently clear and information of the light-absorbing structure. These techniques are useful for developing the noninvasive imaging of the light- absorbing structure. It is useful for developing the di- agnosis modality and the application in medical field. ACKNOWLEDGMENTS This presented research was supported by Ho Chi Minh City University of Technology, VNU-HCM un- der Grant T-KHUD-2018-83. CONFLICT OF INTEREST The authors declare no conflict of interest. SI19 Science & Technology Development Journal – Engineering and Technology, 3(SI3):SI10-SI21 Figure17: Effectiveness of scattering suppressionusing correctly estimateddepth. The yellowdashed-line square represents the true size of the absorber. AUTHORS’ CONTRIBUTIONS Conceptualization: Trung Nghia Tran, Takeshi Na- mita, and Koichi Shimizu. Methodology: Trung Nghia Tran, Kohei Yamamoto, and Takeshi Namita. Software: Trung Nghia Tran, Kohei Yamamoto, Ngoc An Dang Nguyen, and Minh Quang Nguyen. Validation: Trung Nghia Tran, Kohei Yamamoto, Ngoc An Dang Nguyen, and To Ni Phan Van. Data curation: Ngoc An Dang Nguyen, and To Ni Phan Van Writing—original draft preparation: Ngoc An Dang Nguyen, and To Ni Phan Van. Writing—review and editing: Anh Tu Tran, and Trung Nghia Tran. Supervision: Trung Nghia Tran, Takeshi Namita, and Koichi Shimizu. All authors have read and agreed to the published ver- sion of the manuscript. REFERENCES 1. Tobisawa N, Namita T, Kato Y, Shimizu K. Injection Assist Sys- tem with Surface and Transillumination Images. 5th Interna- tional Conference on Bioinformatics and Biomedical Engineer- ing, Wuhan. 2011;p. 1–4. Available from: https://doi.org/10. 1109/icbbe.2011.5780365. SI20 Science & Technology Development Journal – Engineering and Technology, 3(SI3):SI10-SI21 2. Shimizu K, Tochio K, Kato Y. Improvement of transcutaneous fluorescent images with a depth-dependent point-spread function. Appl Opt. 2005;44:2154–2161. PMID: 15835361. Available from: https://doi.org/10.1364/AO.44.002154. 3. Tran TN, Yamamoto K, Namita T, Kato Y, Shimizu K. Three- dimensional transillumination image reconstruction for small animal with new scattering suppression technique. Biomed Opt Express. 2014;5(5):1321–1335. PMID: 24876998. Available from: https://doi.org/10.1364/BOE.5.001321. 4. Tran TN, Van TNP, Tran AT, Yamamoto K, Namita T, Shimizu K, et al. Near -infrared transillumination imaging of veins us- ing low -cost camera and scattering suppression - validation of practicality of developed system. Journal of Development Sci- ence and Technology. 2017;20(K3/2017):67–73. Available from: https://doi.org/10.32508/stdj.v20iK3.1094. SI21
File đính kèm:
depth_estimation_of_the_absorbing_structure_in_a_slab_turbid.pdf